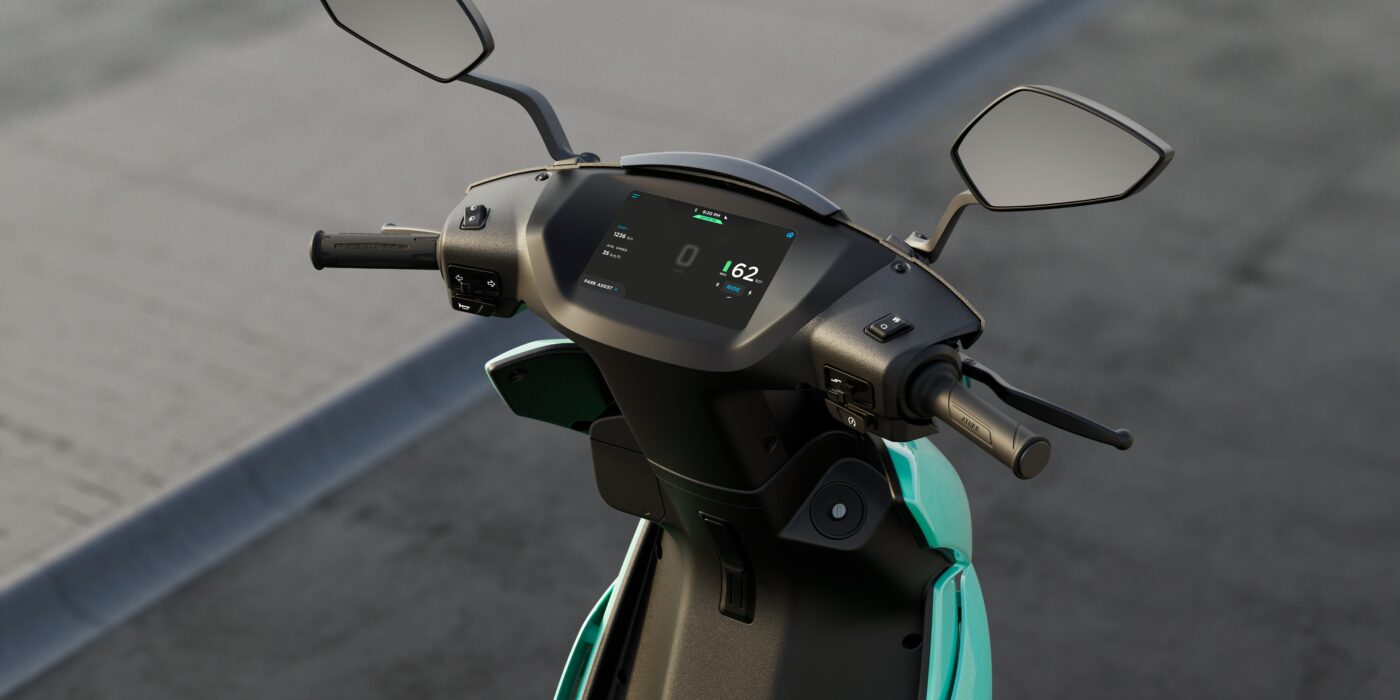
Overview
Electrification of transport is a central pillar of the much-needed emission reductions in the transport sector, along with a strong shift toward public transport, walking, and cycling. It will require a substantial scaling up of renewable electricity to contribute to the decarbonization of the energy and transport sectors. While decarbonized economies have great benefits, the transition to get there holds some challenges.
Low- and middle-income countries (LMICs) encounter more pronounced challenges, as they often face substantial development needs in the intertwined power and transport sectors. While countries make choices on how they use their resources, developing renewable electricity and e-mobility together offer synergies that allow investments to be optimized rather than allow for compromises.
It is important to explore the interdependencies and synergies of developing e-mobility and renewable electricity. The three articles presented in this paper address the relevance of sector modeling to inform long-term strategies for decarbonizing energy and transport; the opportunities of integrating road transport electrification and the development of renewable electricity; and the potential synergies between railway electrification and grid expansion. They illustrate examples from Latin America, Africa, and Asia.
Publications with the same themes
Publications with the same study countries
PDF content (text-only)
GRA IN ACTION SERIES ELECTROMOBILITY AND RENEWABLE ELECTRICITY Developing Infrastructure for Synergies © 2022 SuM4AllTM Internet: http://www.sum4all.org Standard Disclaimer This publication was produced by the Sustainable Mobility for All (SuM4AllTM) initiative. The findings, interpretations, and conclusions expressed in this paper do not necessarily reflect the views of the SuM4All member organizations or the governments they represent. SuM4All does not guarantee the accuracy, completeness, or currency of the data included in this work and does not assume responsibility for any errors, omissions, or discrepancies in the information, or liability with respect to the use of or failure to use the information, methods, processes, or conclusions set forth. The boundaries, colors, denominations, and other information shown on any map in this work do not imply any judgment on the part of SuM4All or its members concerning the legal status of any territory or the endorsement or acceptance of such boundaries. Nothing herein shall constitute or be considered to be a limitation upon or waiver of the privileges and immunities of SuM4All, all of which are specifically reserved. Rights and Permissions This work is available under the Creative Commons Attribution 3.0 IGO license (CC BY 3.0 IGO) http://creativecommons.org/licenses/by/3.0/igo. Under the Creative Commons Attribution license, you are free to copy, distribute, transmit, and adapt this work, including for commercial purposes, under the following conditions: Attribution—Please cite the work as follows: Sustainable Mobility for All. 2022. Electromobility and Renewable Electricity: Developing Infrastructure for Synergies. Washington DC, ISBN: 979-8-9860188-5-0. License: Creative Commons Attribution CC BY 3.0 IGO Translations—If you create a translation of this work, please add the following disclaimer along with the attribution: This translation was not created by Sustainable Mobility for All and should not be considered an official Sustainable Mobility for All translation. Sustainable Mobility for All shall not be liable for any content or error in this translation. Adaptations—If you create an adaptation of this work, please add the following disclaimer along with the attribution: This is an adaptation of an original work by Sustainable Mobility for All. Views and opinions expressed in the adaptation are the sole responsibility of the author or authors of the adaptation and are not endorsed by Sustainable Mobility for All. Third-party content—Sustainable Mobility for All does not necessarily own each component of the content contained within the work. Sustainable Mobility for All, therefore, does not warrant that the use of any third-party-owned individual component or part contained in the work will not infringe on the rights of those third parties. The risk of claims resulting from such infringement rests solely with you. If you wish to re-use a component of the work, it is your responsibility to determine whether permission is needed for that re-use and to obtain permission from the copyright owner. Examples of components can include but are not limited to, tables, figures, or images. All queries on rights and licenses should be addressed to Sustainable Mobility for All, e-mail: sum4all@worldbankgroup.org. ISBN: 979-8-9860188-5-0 ELECTROMOBILITY AND RENEWABLE ELECTRICITY Developing Infrastructure for Synergies ii CONTENTS ABBREVIATIONS . . . . . . . . . . . . . . . . . . . . . . . . . . . . . . . . . . . . . . . . . . IV FOREWORD . . . . . . . . . . . . . . . . . . . . . . . . . . . . . . . . . . . . . . . . . . . . . . . V ACKNOWLEDGMENTS . . . . . . . . . . . . . . . . . . . . . . . . . . . . . . . . . . . . . . VI EXECUTIVE SUMMARY . . . . . . . . . . . . . . . . . . . . . . . . . . . . . . . . . . . . . . . 1 INTRODUCTION . . . . . . . . . . . . . . . . . . . . . . . . . . . . . . . . . . . . . . . . . . . . 3 Background . . . . . . . . . . . . . . . . . . . . . . . . . . . . . . . . . . . . . . . . . . . . . . . . 3 Objectives . . . . . . . . . . . . . . . . . . . . . . . . . . . . . . . . . . . . . . . . . . . . . . . . . 3 Content and structure . . . . . . . . . . . . . . . . . . . . . . . . . . . . . . . . . . . . . . . 4 Related work under SuM4All . . . . . . . . . . . . . . . . . . . . . . . . . . . . . . . . . . 4 1. SECTOR MODELING TO INFORM LONG-TERM STRATEGIES FOR DECARBONIZING ENERGY AND TRANSPORT: EXPERIENCES FROM LATIN AMERICA AND THE CARIBBEAN REGIONS . . . . . . . . 5 Key takeaways . . . . . . . . . . . . . . . . . . . . . . . . . . . . . . . . . . . . . . . . . . . . . . 5 Introduction . . . . . . . . . . . . . . . . . . . . . . . . . . . . . . . . . . . . . . . . . . . . . . . 6 Modeling and stakeholder engagement for better long-term strategies . . . . . . . . . . . . . . . . . . . . . . . . . . . . . . . . . . . . . . . . . . . . . . . . . 6 Assessing the fiscal impact and the need for reforms as part of long-term strategies . . . . . . . . . . . . . . . . . . . . . . . . . . . . . . . . . . . . . . . 8 2. ROAD TRANSPORT ELECTRIFICATION AND RENEWABLES: OPPORTUNITIES FOR INTEGRATION . . . . . . . . . . . . . . . . . . . . . . . 11 Key takeaways . . . . . . . . . . . . . . . . . . . . . . . . . . . . . . . . . . . . . . . . . . . . . . 11 Road transport and renewable electricity’s potential for sustainable and low-carbon development . . . . . . . . . . . . . . . . . . . . . . . . . . . . . . . . . . . . . 12 Trends and challenges in electromobility in LMICs . . . . . . . . . . . . . . . . . 12 Electromobility impact on electricity supply . . . . . . . . . . . . . . . . . . . . . . 14 Trends in Renewable Electricity . . . . . . . . . . . . . . . . . . . . . . . . . . . . . . . . 16 Synergies of Renewable Electricity and Electromobility . . . . . . . . . . . . . 17 Opportunities for growing electromobility and renewable electricity in synergy . . . . . . . . . . . . . . . . . . . . . . . . . . . . . . . . . . . . . . . . . . . . . . . . . . . 18 Actions for integrated development of both sectors . . . . . . . . . . . . . . . 26 3. EXPLORING THE POTENTIAL SYNERGIES BETWEEN RAILWAY ELECTRIFICATION AND GRID EXPANSION . . . . . . . . . . . . . . . . . . . 30 Key takeaways . . . . . . . . . . . . . . . . . . . . . . . . . . . . . . . . . . . . . . . . . . . . . 30 Railways as an energy-efficient and high-volume mode of transport . . 31 Railway’s low carbon energy options . . . . . . . . . . . . . . . . . . . . . . . . . . . 31 Challenges and synergies of direct rail electrification . . . . . . . . . . . . . . 31 Experiences in the Global South . . . . . . . . . . . . . . . . . . . . . . . . . . . . . . 32 ELECTROMOBILITY AND RENEWABLE ELECTRICITY: DEVELOPING INFRASTRUCTURE FOR SYNERGIES • iii AC Alternating Current BESS Battery Energy Storage System BEV Battery Electric Vehicle CaaS Charging-as-a-Service DFI Development Finance Institutions DT Distribution Transformer EV Electric Vehicle EDR Ethio-Djibouti Railway FAME Faster Adoption and Manufacturing of Electric and Hybrid Vehicles GHG Greenhouse Gas GDP Gross Domestic Product GST Goods and Service Tax ICE Internal Combustion Engine IPP Independent Power Producer LAC Latin America and the Caribbean kW Kilowatt LATAM Latin America LMIC Low- and Medium-Income Countries LT-LEDS Long-Term Low Emissions Development Strategies LTS Long-Term Strategies MDB Multilateral Development Bank NEMMP National Electric Mobility Mission Plan PCS Public Charging Station PPA Power Purchase Agreement RESCO Renewable Energy Service Company RPO Renewable Purchase Obligations SRT Solar Rooftop ToD Time of Day ToU Time of Use VGI Vehicle-Grid-Integration V2X Vehicle-to-Everything ZEV Zero Emission Vehicle ABBREVIATIONS iv • Abbreviations ELECTROMOBILITY AND RENEWABLE ELECTRICITY: DEVELOPING INFRASTRUCTURE FOR SYNERGIES • v FOREWORD S ince its inception in 2017, the Sustainable Mobility for All (SuM4All) Partnership has been advocating for sustainable mobility in international forums, with transport decarbonization being one of the four goals for transport systems to achieve globally. The Partnership’s 56 Member organizations were instrumental in framing the policy knowledge around decarbonization, universal access, efficiency, and safety that would support cities and countries on the path to achieving these goals. In recognition of the Partnership's achievements, the United Nations Climate Change Conference (UNFCCC) in 2021 acknowledged the Partnership as a champion of transport decarbonization in climate change action around the world. The 27th United Nations Climate Change Conference (COP27) will take place in Egypt between November 6 and 18, 2022. COP27 is expected to advance action on several fronts— adaptation, the just transition toward decarbonization, and climate finance. Early in 2022, the Partnership engaged with country decision makers at a roundtable discussion to identify the most pressing demands for action on transport to bring forward at COP27. Appropriate e-mobility choices, international cooperation, investing in public transport, and the business of exporting used vehicles to the Global South, were the issues that took center stage. The Partnership mobilized itself into working groups to delve deep into these issues, develop a clear policy agenda for action at COP27, and influence policy debates on these matters. We are pleased to release five important contributions to COP27 in the “GRA in Action Series.” They include: (i) E-mobility in Low-Income Countries in Africa: Finance, Governance, and Equity; (ii) Decision-Making Tool for E-Mobility Investments; (iii) Electromobility and Renewable Electricity: Developing Infrastructure for Synergies; (iv) Empowering E-mobility in the Global South: The Case of Two Cities–Cuenca and Nairobi; and (v) and Key Strategic Areas of Action to Address in Unlocking Public Transport’s Potential to Deliver on Climate and Sustainable Development. The products are the outcome of a year of engagement with Member organizations, countries' decision makers, partners, and field experts. The "GRA in Action Series" aims at generating a better understanding of transport decarbonization, collecting global experiences, and deep diving into the associated policy measures in the Global Roadmap of Action (GRA) to make them more pragmatic for countries' decision makers. We thank the Sustainable Energy for All (SEforAll), the UK’s High Volume Transport Applied Research (HVT) and the Climate Compatible Growth (CCG) Programme, the Transformative Urban Mobility Initiative (TUMI), and the International Association of Public Transport (UITP), for leading the preparation of this important policy paper on Developing Infrastructure for Synergies between Electromobility and Renewable Electricity. Focusing on Low- and Middle-Income Countries (LMICs), this paper provides guidance on coordination needs between transport and energy decision-makers. Sustainable Mobility for All Steering Committee (On behalf of our 56 Member organizations) November 2022, Washington, D.C. Under the umbrella of the Sustainable Mobility for All (SuM4All) partnership, two working groups “Energy and Mobility”, co-led by Ben Hartley (SEforAll), Bernard Obika (HVT) and Stefanie Sohm (HVT/CCG), and “E-mobility”, co-led by Marvin Stolz (TUMI) and Philip Turner (UITP) prepared this paper. Other co-authors who contributed to this paper are listed chronologically: Jairo Quirós-Tortós and Luis F. Víctor Gallardo (University of Costa Rica); Rahul Bagdia, Yamini Keche, Kunjan Bagdia and Ankit Agrawal (pManifold Consultants) with the support of Gireesh Shrimali, Abhishek Ranjan, and Chandrasekhar Reddy Atla; and Marcelo Blumenfeld (University of Birmingham). The paper also benefitted from inputs of working group members: Suzanne Spooner (AFD), Benjamin Jeromin (BMZ), Jaime Ruíz Huescar (Cities Forum), Shailendra Kaushik (Cities Forum), Jill Warren (ECF), Stefano Ammirati (FIA), Marc Juhel (FIA), Sheila Watson (FIA Foundation), Justin Coetzee (GoMetro), Holger Dalkmann (HVT/CCG), Maha Mousavi (ICAO), Chrystelle Damar (ICAO), Thilly de Bodt (ICAO), Geert van Waeg (IFP), Susanna Zammataro (IRF), Mohammed Alsayed (IsDB), Ahmed Al Qabany (IsDB), Jacob Mason (ITDP), Angelika Zwicky (KfW), Reiner Koblo (KfW), Arto Keklikian (NCC Canada), Nyaga Kebuchi (STA), Maruxa Cardama (SLOCAT Partnership), Nikola Medimorec (SLOCAT Partnership), Mark Major (SLOCAT Partnership), Laura Menzel (TUMI), Insa Illgen (TUMI), Armin Wagner (TUMI), Ha Joo Uyun (UIC), Lucie Anderton (UIC), Francesco Dionori (UNECE), George Georgiadis (UNECE), Fadiah Ahmadi (UNESCAP), Wei-Shiuen Ng (UNESCAP), Anne Joselin (UK FCDO), Sergio Avelleda (WRI), Ben Welle (WRI), Fatima Arroyo Arroyo (WB), Gurpreet Singh Sehmi (WB), Mary Ngaratoki Fabian (WB), Josephine Njoki Irungu (WB), and Urska Skrt (WBCSD). We acknowledge the guidance received from the SuM4All Steering Committee comprising Benjamin Jeromin (BMZ), Anne Joselin (FCDO), Sheila Watson (FIA Foundation), Susanna Zammataro (IRF), Atiq Ahmad (IsDB), Alana Dave (ITF), Nicolas Beaumont (Michelin), Ben Hartley (SEforALL), Maruxa Cardama (SLOCAT), Marvin Stolz (TUMI), Jan Hoffman (UNCTAD), Francesco Dionori (UNECE), Jim Walker (Walk21), Benjamin Welle (WRI), Nicolas Peltier (World Bank), and Binyam Reja (World Bank). We thank the SuM4All Secretariat for their support, including Nancy Vandycke, Mary Ngaratoki Fabian, Josphine Njoki Irungu, Gurpreet Singh Sehmi, and Emiye Gebre Egziabher Deneke. Chitra Arcot was the principal editor, and Duina Reyes designed the report. This paper is part of the GRA in Action Series funded by the World Bank, the German Federal Ministry for Economic Cooperation and Development (BMZ), the Foreign, Commonwealth and Development Office (FCDO), and the Michelin Foundation. This work was funded by UKAID through the UK Foreign, Commonwealth & Development Office (FCDO) under the High Volume Transport Applied Research (HVT) Programme, managed by DT Global. HVT undertakes research into sustainable transport development in LICs across Africa and South Asia. ACKNOWLEDGMENTS vi • Acknowledgments EXECUTIVE SUMMARY E lectrification of transport is a central pillar of the much-needed emission reductions in the transport sector, along with a strong shift toward public transport, walking, and cycling. It will require a substantial scaling up of renewable electricity to contribute to the decarbonization of the energy and transport sectors. While decarbonized economies have great benefits, the transition to get there holds some challenges. Low- and middle-income countries (LMICs) encounter more pronounced challenges, as they often face substantial development needs in the intertwined power and transport sectors. While countries make choices on how they use their resources, developing renewable electricity and e-mobility together offer synergies that allow investments to be optimized rather than allow for compromises. It is important to explore the interdependencies and synergies of developing e-mobility and renewable electricity. The three articles presented in this paper address the relevance of sector modeling to inform long-term strategies for decarbonizing energy and transport; the opportunities of integrating road transport electrification and the development of renewable electricity; and the potential synergies between railway electrification and grid expansion. They illustrate examples from Latin America, Africa, and Asia. The following conclusions and suggestions can be summarized from the articles: “Sector modeling to inform long-term strategies for decarbonizing energy and transport: Experiences from Latin America and the Caribbean (LAC) Regions” f Long-term strategies (LTSs) contemplating net zero emissions by 2050 are key instruments in driving transformational changes in countries. f LTSs allow the mapping out of mid- and short-term goals that will help achieve the long-term target. Costa Rica is a good example with a vision of nearly full electrification by 2050 and an intermediate step of 30 percent by 2035 and is piloting electric and hydrogen buses. f Energy modeling and strong stakeholder engagement are critical for increasing the acceptability and transparency in LTS design. f Transformations needed to reach net zero emissions require investments that are outweighed by the benefits that they will bring. f Decarbonizing transport would, in most countries in LAC, cause a fiscal impact due to the high reliance of fuel-related taxes. However, the net benefits of decarbonizing are larger. Crucially, since the fiscal impact occurs in the long ELECTROMOBILITY AND RENEWABLE ELECTRICITY: DEVELOPING INFRASTRUCTURE FOR SYNERGIES • 1 run, governments can execute tax adjustments to eliminate the fiscal impact while maintaining net benefits for all actors. “Road transport electrification and renewables: Opportunities for integration” f Road transport and renewable electricity sectors a considerable opportunity for capturing synergies that can decarbonize and improve access to electricity and transportation. f Improving business models and financial investments in both sectors need suitable integrated policy measures, funding mechanisms, and governance structures to flourish, especially in LMICs with unstable grid infrastructure and limited access to finance. f Actions for effective e-mobility and renewable electricity integration: • Governments: Drive renewable electricity and electric vehicle (EV) demand and supply with a clear vision and a road map toward long-term commitments. • Power utilities: Add renewable electricity to production capabilities and support solar rooftops and smart charging solutions for customers, encouraging the use of renewable electricity for EV charging. • Manufacturers: Build affordable and locally suitable, high quality EVs integrating renewable electricity through efficient and clean supply chains. • Operators: Establish valued, scalable business models that can solve the affordability and productivity challenge. • Financiers: Provide easy access to low cost financing and scalable financing instruments. • Renewable electricity developers: Grow the mix with customers to optimize electricity costs and network efficiency through grid integration. • Power exchanges: Support favorable infrastructure and price mechanisms to allow round the clock renewable electricity and EV integration. “Exploring the potential synergies between railway electrification and grid expansion” Railway transport must take a central part in the decarbonization of the transport sector, as it is more efficient in the use of resources than road-based modes. Reaching the full potential of environmental benefits that railways can bring also depends on the shift away from diesel toward low carbon energy sources. f Railway electrification, the most traditional way to decarbonize operations, poses a significant economic challenge due to the considerable capital investment required. f Challenges related to railway electrification may be accentuated in countries of the Global South. f The synergies between the energy and the railway sector may hold the key to bypass the chicken-and-egg paradigm and unlock the economic justification for such investments. f Railway electrification is only viable when sufficient electricity supply already exists or if the electrification of railways and expansion of the grid can be combined to promote wider transport and electricity access for economic growth. f Electrified railway services create a new and known demand for electricity and the joint development of electrified rail and renewable electricity infrastructure can optimize investments in both sectors. 2 • Executive Summary INTRODUCTION BACKGROUND Electrification of transport is a central pillar of the much-needed emission reductions in the transport sector, along with a strong shift toward public transport, walking, and cycling It will require a substantial scaling up of renewable electricity to contribute to the decarbonization of the energy and transport sectors. Countries can increase their energy independence through locally produced renewable electricity. They can free up resources spent on fossil fuel imports and subsidies for investments in their economies. In the medium- and long-term, countries can benefit from the lower overall cost of a decarbonized economy. Yet, these transitions come with their challenges. Higher shares of intermittent renewables such as wind and solar electricity require a greater grid and storage capacity flexibility. Charging for a growing fleet of electric vehicles (EVs) needs to be managed so that it does not threaten the grid’s stability or compromise other sectors' energy needs. Likewise, electrifying railways comes with large additional capacity requirements. And both sectors, transport, and energy need substantial investments to initiate and accelerate their shift into a low carbon development pathway. Low- and middle-income countries (LMICs) encounter even more pronounced challenges, as they often face substantial development needs in both the power and the transport sector. In the transport sector, the development of electromobility (e-mobility) should be aligned to support other aspects of sustainable transport systems such as accessibility, air pollution, and congestion. In the electricity sector, generation capacity and grids may be insufficient to satisfy already existing needs, and people may still lack access to energy. Providing more people with reliable, clean, and affordable electricity must be at the center of the energy sector’s development effort. While countries make choices on how to use their resources, developing renewable electricity and e-mobility together also offers synergies that allow investments to be optimized rather than allow for compromises. OBJECTIVES Prepared under the umbrella of the Sustainable Mobility for All (SuM4All) partnership and as a joint product of the working groups “Energy and Mobility” and “E-mobility”, this paper intends to raise awareness about the need for more cross-sectoral exchange between transport and energy. The paper also seeks to stimulate and enrich the discussion on the ways both sectors can collaborate and coordinate to help overcome the challenges and make use of the opportunities that e-mobility entails. ELECTROMOBILITY AND RENEWABLE ELECTRICITY: DEVELOPING INFRASTRUCTURE FOR SYNERGIES • 3 The paper aims to inform policy makers at the national and local levels from transport, energy and environment, urban and rural development, and industry. It equally aims to serve utilities, energy producers, public and private transport fleet and infrastructure operators, investors as well as the international finance and development community. The paper's working groups also wish to contribute meaningfully at the United Nations Climate Change Conference 2022 to the much-needed acceleration of climate action in transport and energy. CONTENT AND STRUCTURE In this paper, we explore the interdependencies and synergies of developing e-mobility and renewable electricity. With a focus on LMICs, we discuss joint development of electric ground transport—road vehicles, ranging from two-wheelers to heavy duty vehicles, and rail—and renewable electricity. The paper comprises three independent yet complementary articles: The first article “Sector modeling to inform long-term strategies for decarbonizing energy and transport: Experiences from Latin America and the Caribbean” looks at the necessity and value of modeling and long-term planning as strategic tools to guide decision making in the energy and transport sector. Examples from Latin America and the Caribbean illustrate the benefits and the approaches governments can take. The second article “Road transport electrification and renewables: Opportunities for integration” focuses on the potential to combine developments of e-mobility in the road vehicle sector and expansion of renewable electricity generation. It explores different business models, provides considerations on their benefits, limitations, and suitability for LMICs, and gives recommends how key actors can support their development. The third article “Exploring the potential synergies between railway electrification and grid expansion” sets the focus on rail electrification, and the challenges and opportunities to expand rail and grid networks for mutual benefit. The paper introduces examples from Africa and India. RELATED WORK UNDER SUM4ALL As part of the “GRA in Action” series1 the paper adds to the body of GRA publications dedicated to sustainable electrification of the transport sector. It is the first paper that sets a particular focus on the intersection of transport and energy. Other related papers of the GRA in Action series include: f E-mobility in Low-Income Countries in Africa: Finance, Governance, and Equity (SuM4All 2022) f Empowering the City of Cuenca Towards Transport Decarbonization: A Vision for Electric Mobility (SuM4All 2022) f Empowering E-mobility In the Global South: The Case of Two Cities–Cuenca and Nairobi (SuM4All 2022) f Decision-Making Tool for E-Mobility Investments (SuM4All 2022) f Unlocking Public Transport’s Potential to Deliver on Climate and Sustainable Development: 6 Strategic Areas of Action to Address (SuM4All 2022) f Sustainable Electric Mobility: Building Blocks and Policy Recommendations (SuM4All 2021) f Electromobility in the Global South: An Equitable Transition toward Road Passenger Transport Decarbonization (SuM4All 2021) f The Digital Toolkit for Energy and Mobility (SuM4All 2021) Notes 1. The GRA in Action series contains actionable guidelines developed from the recommendations made in the Global Roadmap of Action toward Sustainable Mobility (GRA) - a Policy Tool developed by the Sustainable Mobility For All (SuM4All) initiative. Drawing from the Catalogue of Policy Measures, these papers take a deep dive into policies and global experience available to enable policymakers to take action on the ground. Available: https://www.sum4all.org/publications/gra-action-series. 4 • Introduction KEY TAKEAWAYS f Long-term strategies (LTSs) contemplating net-zero emissions by 2050 are key instruments in driving transformational changes in countries. f LTSs allow the mapping out of mid- and short-term goals that will help achieve the long-term target. Costa Rica is a good example with a vision of nearly full electrification by 2050 and an intermediate step of 30 percent by 2035 and is piloting electric and hydrogen buses. f Energy modeling and strong stakeholder engagement are critical for increasing the acceptability and transparency in LTS design. In Costa Rica, the Ministry of Environment and Energy led their LTS design with technical support from the University of Costa Rica. Similar examples can be cited from Peru, Chile, Guatemala, and other Latin America and the Caribbean (LAC) countries. f Transformations needed to reach net-zero emissions require investments that are outweighed by the benefits that they will bring. The net benefits in Costa Rica are estimated to be US$41 billion (Groves et al. 2020), US$7 billion in Chile (Benavides et al. 2021) and US$140 billion in Peru (Quirós-Tortós et al. 2021) f Decarbonizing transport would, in most countries in LAC, cause a fiscal impact due to the high reliance of fuel-related taxes. However, the net benefits of decarbonizing are larger. Crucially, since the fiscal impact occurs in the long run, governments can execute tax adjustments to eliminate the fiscal impact while maintaining net benefits for all actors. f Decarbonizing would also bring other benefits not discussed in detail in this article. The LAC region could see a net creation of 15 million jobs in 2030 by speeding up decarbonization and providing the necessary re-skilling (Saget et al. 2020). SECTOR MODELING TO INFORM LONG-TERM STRATEGIES FOR DECARBONIZING ENERGY AND TRANSPORT: EXPERIENCES FROM LATIN AMERICA AND THE CARIBBEAN REGIONS Jairo Quirós-Tortós and Luis F. Víctor Gallardo ELECTROMOBILITY AND RENEWABLE ELECTRICITY: DEVELOPING INFRASTRUCTURE FOR SYNERGIES • 5 1 INTRODUCTION The Paris Agreement calls on countries to devise long-term strategies (LTSs)1 contemplating low greenhouse gas (GHG) emissions (Waisman et al. 2016) to limit the global average temperature rise above pre-industrial levels to well below 2°Celcius, preferably 1.5°Celcius. LTSs should aim to reach and sustain net-zero global anthropogenic carbon dioxide emissions to halt global warming (IPCC 2021). While the world is not on track to reach the 1.5°Celcius temperature limit (Matthews and Wyne 2022), 11 countries in Latin American and the Caribbean (LAC), of 50 worldwide, have already set targets to reach net-zero carbon or GHG emissions (Fazekas et al. 2022). While only six countries in LAC have submitted their LTSs, multilateral development banks (MDBs) are working with governments to design and present their LTSs soon. In many cases, these LTSs are being developed using well-known and established methodologies and robust decision making (Lempert 2019). The LAC region could be an example to other countries on approaches to design LTSs (Calfucoy et al. 2022). Increasing renewable energy generation and reducing fossil fuel consumption from transport are key transformations to achieve net-zero emissions. While the price tag on decarbonizing a country's economy in LAC can range between 7 to 19 percent of their annual gross domestic product (GDP) (Galindo et al. 2022)—about half of it in the energy sector—the benefits of doing so will outweigh the costs, as studies in the region have shown. The net benefits in Costa Rica are estimated to be US$41 billion (Groves et al. 2020), US$140 billion in Peru (Quirós-Tortós et al. 2021), and US$7 billion in Chile (Benavides et al. 2021). The transition to net-zero emissions will also bring challenges. Switching to renewable energy sources and e-mobility will lower government revenue as their tax income is highly dependent on fossil fuels and internal combustion engine (ICE) vehicles. On the other hand, diversifying the economy in the region will open new sources of revenue and bring more jobs. Executing the transformation will require addressing technological, financial, social, and policy challenges. LTSs are an important instrument for guiding action, anticipating and managing trade-offs, and designing policies and investments that enable the transition (IDB & DDPLAC 2019; Victor-Gallardo 2022). We explore two desirable features of LTS elaboration: (i) the importance of modeling and stakeholder engagement when it comes to estimating the impact of sectoral transitions and identifying implementation actions; and (ii) the possible fiscal implications and responses of the decarbonization process in LAC and their link to LTSs. MODELING AND STAKEHOLDER ENGAGEMENT FOR BETTER LONG-TERM STRATEGIES Energy modeling—using mathematical models to generate insights about the future of energy systems— can provide policy support when based on rigorous analytics and good governance (Howells et al. 2021). When applied in conjunction with close stakeholder collaboration and consultations through workshops or bilateral meetings, energy modeling can help actors gain perspective, clarity, and agreement about the transformations needed to reach decarbonization and inform a county’s LTSs. The range of stakeholders varies per country, but generally, energy modeling involves the ministers of energy, environment, transport, finance, and planning. Energy modeling must capture the synergies between sectors. Without this sector coupling, the models may not be able to represent the true transformation needed. For instance, modeling electricity and transport sectors together can lead to understanding power grid requirements from ongoing electrification of the vehicle fleet. Thus, combined sector modeling for energy and transport necessitates engaging a country’s transport stakeholders. For example, the design of the energy component of the National Decarbonization Plan of Costa Rica was led by the Ministry of Environment and Energy and involved stakeholders from the Ministry of Public Works and Transport, the Costa Rican Refinery of Petroleum, the Costa Rican Institute of Rails, members of the eight power utilities, the transmission system operator, civil society, academia, and the private sector. Similar groups were, or are being, created in Chile, the 6 Dominican Republic, Guatemala, Peru, and Uruguay. Close stakeholder engagement also increases modeling transparency and enables good representation of the system under analysis. The LTS-related development opportunities for each country will vary. However, some recommendations for better coupling LTS and energy modeling efforts are: f LTSs respond to international requirements and identify development opportunities for national actors. Without a linkage to a development strategy, an LTS can become irrelevant as an inert policy formality (Calfucoy et al. 2022). For that reason, models must continually improve and address the questions and knowledge gaps of the policymaking community and stakeholders (Howells et al. 2021). Moreover, modeling and LTS targets must be transparent. The cases of Costa Rica and Chile are notable examples. Their LTSs were then used to align all other policy instruments as with the national development plan and increase their respective relevance. In addition, the University of Costa Rica continually improves and updates the models used for the design of energy policy based upon new feedback or requests from stakeholders and international data. f LTS efforts need to increase the understanding of measures needed to achieve the transformations. The measures should link to country-specific opportunities that increase competitiveness (Calfucoy et al. 2022). Hence, alignment with broader socio-ecological objectives and striving for equity should be LTS features (Fankhauser et al. 2022). Once again, the case of Chile and Costa Rica are outstanding examples. Their energy transition modeling highlights key transformations with relatively low investment and high benefits that could then be used to promote other policies. f The institutions responsible for implementing LTSs should maintain continuous stakeholder engagement (Calfucoy et al. 2022), supported with rigorous technoeconomic analytics. For instance, the Ministry of Environment and Energy in Costa Rica works closely with the University of Costa Rica to improve the model and provide additional insights continuously. They continue to work to understand the ecosystem needed to enable hydrogen for heavy transport in the country by 2030 and 2050. At this stage, institutions responsible for executing LTSs will define investments and policy reforms that are concrete and viable. While energy modeling offers policy insights for strategic planning, other challenges, such as integrating renewable energy sources and zero emission vehicles (ZEVs), will also have to be dealt with other tools. An example would be those that can facilitate the study of grid operation that includes system balancing and security services. With adequate technologies and tools, the transport sector can support balancing grid operations such as through vehicle-to-grid, smart charging, and dynamic end user pricing to encourage users to charge and provide their electric vehicle (EV) battery in line with the grid’s balancing needs. Energy modeling can help understand this integration. Countries in LAC have used models to primarily study their electricity systems. Integrated models were adopted to understand the synergies between systems, such as electricity and transport, electricity and gas, and electricity, transport, and gas. Chile Costa Rica, and Peru used integrated models to investigate the transformations needed to achieve net-zero emissions by mid-century (Benavides et al. 2021; Groves et al. 2020; Quirós-Tortós et al. 2021). Some countries are working on the development of models to support the creation of their LTSs, including the Dominican Republic, Ecuador, Guatemala, Honduras, Panama, and Uruguay. These models are commonly developed by local universities with international collaboration including the financial support of MDBs. They are built using local data and stakeholder engagement. While some countries use licensed software for developing their LTSs, there is an ongoing appetite in the region to move toward open source and free tools (Allington et al. 2021) as these increase transparency, lead to co-development with the international community, and reduce costs. ELECTROMOBILITY AND RENEWABLE ELECTRICITY: DEVELOPING INFRASTRUCTURE FOR SYNERGIES • 7 ASSESSING THE FISCAL IMPACT AND THE NEED FOR REFORMS AS PART OF LONG-TERM STRATEGIES Transport decarbonization reduces the overall economic cost of the transport sector for societies but substituting ICE vehicles for ZEVs erodes a category of fiscal revenues that governments have relied on for decades (Rodriguez et al. 2021; van Dender 2019). In the LAC region, taxes on energy and transport activities amount to one percent of GDP (OECD 2022). Some countries in the LAC region depend on oil and gas revenues for their fiscal revenue as energy producers and exporters (Solano-Rodríguez et al. 2021). In a future where fossil fuels are in less demand and considering decreasing oil and gas reserves, failing to diversify revenue can jeopardize fiscal sustainability and cause stranded asset risks. The COVID-19 pandemic that lowered oil prices in 2020 illustrated this vulnerability. Bolivia, Colombia, Ecuador, Mexico, and Trinidad and Tobago had a fall in government revenue of 0.9, 1.2, 3.4, 0.9 and 3.3 percentage points of GDP respectively in 2020, relative to 2019 (OECD 2022). Governments may face losing direct revenue from the transport sector. But they must contrast this against the possible benefits of transition. They should also account for indirect revenue resulting from non-transport related activities. One metric to measure the benefits of decarbonization are the avoided costs relative to not decarbonizing or a business-as-usual (BAU) scenario. An energy model coupled with a cash transfer estimation model can help understand the overall financial impact of decarbonizing the transport sector. For example, in Costa Rica—a country with an early LTS—the benefits of road transport decarbonization were estimated using modeling tools. An integrated model on the open source energy modeling system (OSeMOSYS) that represents the entire electricity, transport, industrial, residential, and commercial sectors was used to evaluate the costs, investments and operations, of two possible pathways: decarbonizing and BAU. When contrasting the two scenarios, it was found that decarbonizing the transport sector would bring a financial benefit of 1.49 percent of GDP—yearly average in the 2023–50 period (Rodriguez et al. 2021. This magnitude corresponds to the cost avoided by decarbonizing—including vehicle purchases, fuel purchases, maintenance, and transport-related taxes— which households and firms could redirect or save. The country then assessed the fiscal impact defined as the tax revenue difference between scenarios of decarbonizing the transport sector. This was done using a transfer estimation model connected with the energy model to capture all transfers between actors: households, firms, retailers, and government. The study shows that as the decarbonization of transport unfolds, mainly in the mid- and long-term, decarbonizing road transport in Costa Rica could cause a fiscal impact or unperceived revenue of 0.41 percent of GDP in the country—yearly average in the 2023–50 period (Rodriguez et al. 2021). In the case of Costa Rica, the good news is that the fiscal impact is lower than the financial benefit, and an opportunity presents itself to reform the tax structure and have a fiscal-neutral decarbonization transformation in the transport sector (Rodriguez et al. 2021). In the future, reforms could include taxing kilometers traveled, property, import, fuel, and electricity. The advantage of taxing distance is that externalities can be managed (van Dender 2019), that is, who drives pays. Also, property taxes can be more progressive, as wealthier individuals owning expensive vehicles would pay higher taxes than low-income citizens (Rodriguez et al.2021). The magnitude of the reform does not need to be significant: the benefits from more efficient energy and transport systems can trigger higher economic activity, increasing the tax base from the rest of the economy. Owing to this effect and the new industries and business models, the LAC region could see a net creation of 15 million jobs in 2030 by speeding up decarbonization and providing the necessary re-skilling (Saget et al. 2020). Construction and manufacturing jobs would produce the most jobs (UNEP 2019). The possibility of fiscal and job sustainability through decarbonization policies will make them viable. 8 Notes 1. Officially long-term low emissions development strategies (LT-LEDS). References Allington, L., Cannone, C., Pappis, I., Cervantes Barron, K., Usher, W., Pye, S., Brown, E., Howells, M., Zachau Walker, M., Ahsan, A., Charbonnier, F., Halloran, C., Hirmer, S., Cronin, J., Taliotis, C., Sundin, C., Sridharan, V., Ramos, E., Brinkerink, M., … To, L. S. 2022. Selected ‘Starter kit’ energy system modelling data for selected countries in Africa, East Asia, and South America (#CCG, 2021). Data in Brief 42, 108021. https://doi. org/10.1016/J.DIB.2022.108021. Benavides, C., Cifuentes, L., Díaz, M., Gilabert, H., Gonzales, L., González, D., Groves, D., Jaramillo, M., Marinkovic, C., Menares, L., Meza, F., Molina, E., Montedónico, M., Palma, R., Pica, A., Salas, C., Torres, R., Vicuña, S., Miguel Valdés, J., and Vogt-Schilb, A. 2021. (Spanish). Opciones para lograr la carbono-neutralidad en Chile: una evaluación bajo incertidumbre. http://dx.doi.org/10.18235/0003527. Calfucoy, P., Gunfaus, M. T., Fazekas, A., and Vogt-Schilb, A. 2022. Long-Term Strategies for Decarbonization in Latin America: Learnings from Actor-Based Insights into the Drafting Process Inter-American Development Bank Climate Change and Sustainable Development Sector. http://dx.doi.org/10.18235/0004357. Fankhauser, S., Smith, S. M., Allen, M., Axelsson, K., Hale, T., Hepburn, C., Kendall, J. M., Khosla, R., Lezaun, J., Mitchell-Larson, E., Obersteiner, M., Rajamani, L., Rickaby, R., Seddon, N., & Wetzer, T. 2022. The meaning of net zero and how to get it right. In Nature Climate Change 12, 1: 15–21. Nature Research. https://doi. org/10.1038/s41558-021-01245-w. Fazekas, A., Bataille, C., and Vogt-Schilb, A. 2022. Achieving Net-Zero Prosperity: How Governments Can Unlock 15 Essential Transformations. https://doi.org/10.18235/0004364. Galindo, L. M., Hoffman, B., and Vogt-Schilb, A. 2022. How Much Will It Cost to Achieve the Climate Goals in Latin America and the Caribbean? https://doi.org/http://dx.doi.org/10.18235/0004021. Groves, D. G., Syme, J., Molina-Pérez, E., Calvo, C., Rand, H., Víctor-Gallardo, L., Godínez-Zamora, G., QuirósTortós, J., Saavedra-Gómez, V., and Vogt-Schilb, A. 2020. The Benefits and Costs Of Decarbonizing Costa Rica’s Economy: Informing the Implementation of Costa Rica’s National Decarbonization Plan under Uncertainty. https://doi.org/http://dx.doi.org/10.18235/0002867. Howells, M., Quiros-Tortos, J., Morrison, R., Rogner, H., Blyth, W., Godínez, G., Victor, L. F., Angulo, J., Bock, F., Ramos, E., Gardumi, F., Hülk, L., Institut, R. L., and Peteves, E. 2021. Energy system analytics and good governance -U4RIA goals of Energy Modelling for Policy Support. https://doi.org/10.21203/RS.3.RS-311311/V1. IDB (Inter-American Development Bank) & DDPLAC (Deep Decarbonization Pathways for Latin America and the Caribbean). 2019. Getting to Net-Zero Emissions: Lessons from Latin America and the Caribbean. http://dx.doi. org/10.18235/0002024. IPCC (Intergovernmental Panel on Climate Change). 2021. Summary for Policymakers. In: Climate Change 2021: The Physical Science Basis. Contribution of Working Group I to the Sixth Assessment Report of the Intergovernmental Panel on Climate Change. https://doi.org/10.1017/9781009157896.001. ELECTROMOBILITY AND RENEWABLE ELECTRICITY: DEVELOPING INFRASTRUCTURE FOR SYNERGIES • 9 Lempert, R. J. 2019. Robust Decision Making (RDM). Decision Making under Deep Uncertainty, 23–51. https://doi. org/10.1007/978-3-030-05252-2_2. Matthews, H. D., and Wynes, S. 2022. Current global efforts are insufficient to limit warming to 1.5°C. Science 376(6600): 1404–1409. https://doi.org/10.1126/SCIENCE.ABO3378. OECD (Organisation for Economic Co-operation and Development). 2022. Revenue Statistics in Latin America and the Caribbean 2022. OECD. https://doi.org/10.1787/58a2dc35-en-es. Quirós-Tortós, J., Godínez-Zamora, G., Gerardo De La Torre Ugarte, D., Heros, C., Lazo Lazo, J., Ruiz, E., Quispe, B., Diez Canseco, D., Garro, F., Mora, J., Eguren, L., Sandoval, M., Campos, S., Salmeri, M., Baron, R., Fernandez-Baca, J., Saori Iju Fukushima, A., Saavedra, V., and Vogt-Schilb, A. 2021. Costos y beneficios de la carbono neutralidad en Perú: una evaluación robusta. http://dx.doi.org/10.18235/0003286. Rodriguez, M., Victor-Gallardo, L., Quirós-Tortós, J., Jaramillo, M., and Vogt-Schilb, A. 2021. Impacto fiscal de la descarbonización del transporte en Costa Rica y opciones de política para manejarlo. https://doi. org/10.18235/0003402. Saget, C., Vogt-Schilb, A., and Luu, T. 2020. El empleo en un futuro de cero emisiones netas en América Latina y el Caribe. https://doi.org/10.18235/0002509. Solano-Rodríguez, B., Pye, S., Li, P.-H., Ekins, P., Manzano, O., and Vogt-Schilb, A. 2021. Implications of climate targets on oil production and fiscal revenues in Latin America and the Caribbean. Energy and Climate Change, 2, 100037. https://doi.org/10.1016/j.egycc.2021.100037. UNEP (United Nations Environment Programme). 2019. The opportunity, cost and benefits of the coupled decarbonization of the power and transport sectors in Latin America and the Caribbean. https://wedocs.unep. org/20.500.11822/34532. van Dender, K. 2019. Taxing vehicles, fuels, and road use: Opportunities for improving transport tax practice. 44, 59. https://doi.org/10.1787/e7f1d771-en. Victor-Gallardo, L. F. 2022. Robust energy system planning for decarbonization under technological uncertainty: From "Transport electrification to power system investments". https://www.kerwa.ucr.ac.cr/ handle/10669/87273. Waisman, H., Spencer, T., and Colombier, M. 2016. Long-term low emissions development strategies and the Paris Agreement – Why, what and how? https://www.iddri.org/sites/default/files/import/publications/pb0616_ ts-et-al_lt-leds.pdf. 10 ROAD TRANSPORT ELECTRIFICATION AND RENEWABLES: OPPORTUNITIES FOR INTEGRATION KEY TAKEAWAYS f Road transport and renewable electricity sectors provide considerable opportunity for capturing synergies that can decarbonize and improve access to electricity and transportation. f Improving business models and financial investments in both sectors need suitable integrated policy measures, funding mechanisms and governance structures to flourish, especially in LMICs with unstable grid infrastructure and limited access to finance. f Actions for effective e-mobility and renewable electricity integration: • Governments: Drive renewable electricity and electric vehicle (EV) demand and supply with a clear vision and a road map toward long-term commitments. • Power utilities: Add renewable electricity to production capabilities and support solar rooftops and smart charging solutions for customers, encouraging the use of renewable electricity for EV charging. • Manufacturers: Build affordable and locally suitable, high quality EVs integrating renewable electricity through efficient and clean supply chains. • Operators: Establish valued, scalable business models that can solve the affordability and productivity challenge. • Financiers: Provide easy access to low-cost financing and scalable financing instruments. • Renewable electricity developers: Grow the mix with customers to optimize electricity costs and network efficiency through grid integration. f Power exchanges: Support favourable infrastructure and price mechanisms to allow round the clock renewable electricity and EV integration. Rahul Bagdia, Yamini Keche, Kunjan Bagdia, Ankit Agrawal (pManifold) ELECTROMOBILITY AND RENEWABLE ELECTRICITY: DEVELOPING INFRASTRUCTURE FOR SYNERGIES • 11 2 ROAD TRANSPORT AND RENEWABLE ELECTRICITY’S POTENTIAL FOR SUSTAINABLE AND LOW-CARBON DEVELOPMENT Transport and energy are essential levers for economic growth and social development. At the same time, both sectors are among the biggest emitters of greenhouse gas. Globally, transport contributes 23 percent of all energy-related carbon dioxide emissions, whereas the power industry—including coal, gas, and oil generation—accounts for 40 percent (IEA 2021a). In 2021, energy-related carbon dioxide emissions reached a new record high of 36.3 where transport accounts for (IEA 2022a). Decarbonizing both sectors is key to limiting global warming. In low- and middle-income countries (LMICs), both sectors have yet to grow so they can support their countries’ development needs. In transport, a combination of insufficient public transport systems, poor roads, lack of safe infrastructure for walking and cycling, and low rates of vehicle ownership have resulted in reduced access to services for large parts of the population. In the energy sector, many LMICs continue to have relatively low access rates to electricity. While globally the last two decades have seen some progress, 730 million people still lack access to electricity. Sub-Saharan Africa has low rates with 78 percent of people in urban areas and as meagre as 28 percent of people in rural areas having access to electricity.1 Growth of both sectors, however, can translate into increased fossil fuel dependency and greenhouse gas (GHG) emissions. Making low-carbon electricity and transport a supporting element of a country's sustainable development will help mitigate emissions and spending on fossil fuel technologies that may become a stranded assets well before their end of life. While renewables—notably solar, wind, hydro, and geothermal energy—will play a central role in shifting the power industry to a low-carbon pathway, the electrification of transport has evolved as a key strategy for reducing transport’s carbon dioxide emissions. Renewables and electrification of transport offer several benefits in carbon dioxide reduction and beyond. With electric vehicles being more energy efficient than internal combustion engines, EVs contribute to emission reductions even if the grid is not fully decarbonized. In 95 percent of countries in the Global South, EVs are able to reduce emissions (SuM4All 2021). The cleaner the grid becomes, the higher the emissions savings will be. In addition, renewable electricity and electrified transport increase a country’s energy independence if imported fossil fuels are replaced by nationally produced renewable electricity. Financial resources spent on fossil fuels become available for investments in a country’s own economy. As EVs have zero tailpipe emissions, they contribute to improved air quality and public health. Both renewable energy and electromobility (e-mobility) have the potential to be drivers for national and local economic development and for the creation of green jobs. Yet, these transitions come with their challenges. Electrification of transport will create a new demand for which the power sector will have to cater without compromising other electricity needs. Similarly, e-mobility must fill gaps in access rather than widen them. And both sectors require substantive investments. Using synergies of renewable electricity and e-mobility can be an important opportunity to develop both sectors in parallel, and with greater efficiency of public and private financial resources. In the following section, we investigate the potential synergies for integrating electric vehicles across different segments of road transport with renewable energy. We examine different business models with potential for adoption in LMICs and recommend how to support their deployment. TRENDS AND CHALLENGES IN ELECTROMOBILITY IN LMICS The transport sector and its modal share in LMICs are, in general and for reasons of low affordability, less car-centric than in high-income countries. For example, in Asia–Pacific nations like India, the Philippines, Indonesia, and Thailand, two-wheeler scooters and motorcycles are popular modes of transportation (Goldstein Market Intelligence 2021). Across LMICs, paratransit—a form of shared transport 12 services offered by private operators of minibuses, motorcycles, three-wheelers, or non-motorized pedal bikes—play a significant role and fill in gaps of insufficient or even non-existent public transport systems. In South Africa, among motorized road vehicles, cars and taxis have become the prominent mode of transportation (Department of Statistics South Africa 2021). Local circumstances, such as mode shares, cost advantages, and affordability, regulations and incentives, availability of charging, as well as suitable EV models on the market, largely determine the trends and the potential for EV adoption. EV sales are increasing globally and across segments. Nearly 10 percent of global car sales were electric in 2021, sales of electric two- and three-wheelers reached 10 million, and the global electric bus stock is at 670,000 (IEA 2022b. However, their adoption in LMICs is still low, with the exception of China as the leading EV market (tables 2.1 and 2.2). TABLE 2.1. STATE OF EV ADOPTION ELECTRIC 2- AND 3-WHEELERS ELECTRIC 4-WHEELERS ELECTRIC BUSES China dominates with about 57 million electric bicycles sold in 2021 and a total stock of 300 million on the roads (Bloomberg 2021). In many LMICs, electric 2- and 3-wheelers are not registered, so official data are difficult to obtain. However, large fleets of such vehicles have been reported in Bangladesh (e-bikes = 0.6 million; e-rickshaws = 0.5 million) (IDCOL 2019), India, Nepal, and Vietnam (262,000 e-2 wheelers). China leads the e-car market with a share of 50% of the global e-car sales Latin America registered nearly 25,000 EVs sold in 2021, more than doubling the 2020 level. From Mexico to Chile, EVs were 0.7% of total car sales in 2021, with rates varying by country. Brazil saw the most units sold, with 13,000, and Costa Rica led on a percentage basis with 2.7% of all cars sold with a plug. Mexico is the only LATAM country that manufactures EVs, which it also targets as exports to the U.S (BloombergNEF 2022). In May 2020, more than 420,000 electric buses were in China, about 99% of the global fleet As of April 2022, 3,209 electric buses operated across Latin America (Sustainable Bus 2022). Additionally, electric trolley buses are being deployed in many Asian countries including Armenia, China, Kazakhstan, and Mongolia. Indonesia expects 20% EV sales by 2025 and has decreased EV taxes and eliminated BEV luxury taxes in preparation. Customs taxes and levies have been reduced to aid auto and battery manufacturing. South Africa accounted for 0.04% of car sales. By 2050, 50% of Cape Town's automobiles will be electric vehicles. The South African Master Plan (2021–2035) aims to produce 1% of the world's EVs in South Africa. India has established demand and supply side strategies for EV adoption, including FAME (Faster Adoption and Manufacturing of Hybrid and Electric Vehicle), NEMMP (National Electric Mobility Mission Plan), and production linked incentive. Malaysia has plans to establish 125,000 charging stations by 2030. Thailand has introduced a long-term EV policy with the aim of having 1.2 million operational EVs by 2036 and 690 charging stations. Pakistan plans to capture 30% of car and truck sales by 2030 and 90% by 2040. It aims for 50% of 2- and 3-wheeler sales by 2030 and 90% by 2040. Sri Lanka plans to replace all state-owned vehicles with electric or hybrid models by 2025 and all private vehicles by 2040. Zambia aims to capitalize on the growing EV market by increasing its Cobalt production and refinery industries, thereby aiding the overall EV battery production. Nepal announces plans to replace all fuel-based vehicles with EVs by 2030. Fiji intends to fully operate EVs by 2030, at the earliest. TABLE 2.2. EV ANNOUNCEMENTS OF SELECTED COUNTRIES TO DEVELOP E-MOBILITY Source: SLOCAT,2020 ELECTROMOBILITY AND RENEWABLE ELECTRICITY: DEVELOPING INFRASTRUCTURE FOR SYNERGIES • 13 With the adoption of e-mobility, LMICs face specific local challenges in addition to the common challenges faced by all countries. Among the common challenges are the continued higher cost of EVs and the development of charging infrastructure. These may be exacerbated in LMICs due to their limited financial resources. Additional local challenges may relate to an insufficient and unreliable electricity supply, which could, with increased demand from EVs, widen the energy poverty gap. As well, the eventual and relatively high cost of electricity compared to household income—similar to the high cost of fossil transport fuels in many LMICs—poses a barrier to the adoption of e-mobility. Lastly, transport in general is not sufficiently available or affordable for many people. E-mobility can be an opportunity for the adoption of less expensive and more efficient modes of transportation. With greater energy efficiency, the operational cost of EVs is lower than for ICEs. If the gap in upfront cost is reasonable, this will lead to lower cost of e-mobility overall. For example, in many African markets, electric motorcycles are already commercially attractive (Toukam 2022). E-bikes have conquered the Chinese market (Suzhou Shengyi Motor Co., Ltd., SHIMANO INC) and are gaining market share in India (Okinawa, Hero Electric) and other countries as they are more affordable than larger vehicles. E-bikes come with additional benefits of access and mode shift as they allow longer distances to be covered more comfortably and reduce car dependency. Similarly, shared mobility services that provide transport for a greater number of people or goods are more cost-efficient modes of e-mobility. The transition to e-mobility should therefore be accompanied by nationally and locally appropriate policies and priorities. Without planning and investment principles that focus on access, and without considering the respective context, electrification of transport may lead to setbacks for LMICs, especially their lower income groups. ELECTROMOBILITY IMPACT ON ELECTRICITY SUPPLY The ability to electrify road transport in LMICs is also determined by the power sector’s capacity to provide reliable electricity at an affordable cost. With access to electricity as well as sufficient and reliable supply still being a concern in many LMICs, integrated planning and the joint development of both sectors becomes even more important. Beyond the need for additional capacity and grid extension, two typical challenges of the electricity sector in LMICs are grid reliability and high losses in transmission and distribution. They often result in either higher consumer prices or higher public expenses to cover utilities’ forgone revenue. In some countries like Benin, Haiti, Togo, and the Republic of Congo these losses are above 40 percent. Losses can be exacerbated with increased load because of EV charging. Grid reliability is another critical factor and may pose a challenge if many EVs are being charged at the same time. The experience of countries with advanced adoption of EVs shows that users charge vehicles predominantly during night hours from 8 p.m. to 4 a.m. and afternoon hours from 11 a.m. to 4 p.m. Figure 2.1 illustrates the impact of EV charging on the peak power demand of a distribution company in New Delhi, India and the electricity demand from 10,000 EVs —which include two-, three- and four-wheelers— and 100 e-buses in summer (Das 2020). It shows that the EV charging load curve exceeds the demand load curve without EV charging by approximately seven percent. Night charging can be attributed to home charging preferences, while afternoon charging can be attributed to workplace and public charging. EV charging loads are anticipated to be very dynamic, with spikes in the demand curve. This can impact the distribution network, especially in distribution areas with low available hosting capacity, leading to issues such as voltage instability, harmonic distortion, power losses, and unreliable supply. Similar issues with renewable electricity are discussed in the next section. Therefore, good monitoring of connected loads 14 is required at the distribution and transmission level (box 2.1). The impact on the grid can be minimized by introducing discounted tariffs for charging during non-peak hours like time- of use (ToU) or time of day (ToD). Additionally, other EV charging management solutions like battery energy storage system (BESS), smart charging, and vehicle-grid integration (VGI) can be used to mitigate the negative impacts of uncontrolled EV charging. In the early stages of EV adoption, grid stability may not be a major problem for utilities due to the limited number of EVs. However, as EV penetration increases, it can pose considerable risks. To reduce these risks, load balancing between EV loads and other linked loads should be prioritized. The expected growth of demand for both transport and electricity require integrated policy actions like (i) mandating deployment of smart meters and allowing commercial use of EVs for grid services; (ii) collaborative planning for electricity demand and infrastructure; and (iii) investment in both electricity and transport. But e-mobility, as a productive load, is also a new business case for the electricity sector. It gives utilities and independent power producers (IPPs) the opportunity to serve a new demand that can act as a base load and strengthen the financial viability of investments in grid expansion and additional generation capacity. FIGURE 2.1. SUMMER DAILY AVERAGE EV CHARGING LOAD OF A DISTRIBUTION COMPANY IN NEW DELHI. 20 70 120 170 220 270 320 370 1 2 3 4 5 6 7 8 9 10 11 12 13 14 15 16 17 18 19 20 21 22 23 24 MW Hours Daily load curve on a normal day EV charging load Source: Alliance for an Energy Efficient Economy (AEEE) BOX 2.1: EXAMPLE OF INCREASED LOAD AS A RESULT OF EVS Consider a small household with basic lighting and cooling requirements for which the load sanctioned by the utilities was 5 kilowatts (kW). Now, assume that this household has a new power requirement, which is to charge an electric two-wheeler. For fast charging of an electric two-wheeler, a typical charger power rating is 3 kW. As a result of this charge, the load requirement has increased from 5 kW to 8 kW which is 160% of the original load sanctioned by the utilities. Similarly, for slow charging of electric four wheelers, a typical charger power rating is 7 kW. The load requirement owing to this charge would increase from 5 kW to 12 kW which is 240% of the original load sanctioned by the utilities. This additional load because of EV charging may be difficult to monitor by utilities at a distribution transformer (DT) level. In simpler words, each EV (electric 2-, 3-, or 4-wheelers) connected for charging is the equivalent of one new household connection. In such a scenario, it is advisable that load extension should be considered by the consumer; however, in LMICs, consumers may be unwilling to apply for load extension due to associated costs and efforts. Therefore, proper guidelines need to be notified and implemented by the utilities for EV connections to manage grid supply and stability. ELECTROMOBILITY AND RENEWABLE ELECTRICITY: DEVELOPING INFRASTRUCTURE FOR SYNERGIES • 15 It is also an opportunity for the electricity sector to participate in the industry with new business models along the EV value chain. For example, EV manufacturers can deploy renewable electricity at their facilities, operators, and service providers at charging stations, among others. With this, the entire EV value chain from manufacturing to recycling can be further decarbonized by using renewable electricity sources. The smart integration of energy storage systems with renewables such as solar and wind can increase the grid’s flexibility, minimize fixed demand charges, and make business propositions more attractive by supplying consumers with low carbon, reliable, and affordable energy. These types of collaborations between the power and the transport sector would also contribute to improving electricity availability, particularly in rural areas, where the development of renewable-powered microgrid solutions may be coupled with new demand for electric vehicles. TRENDS IN RENEWABLE ELECTRICITY Since 2010, power consumption in LMICs has increased by approximately 4.5 percent per year. According to estimates, the generation of renewable electricity has nearly doubled over the past decade, resulting in 6.6 percent annual growth. Hydropower has been the primary renewable electricity source, followed by solar and wind. Solar has the potential to be the greatest source of renewable electricity generation by 2030. LMICs provided about 8.2 percent of worldwide renewable electricity generation in 2021 (Our World in Data 2021). Globally, solar, wind, hydro, geothermal, wave, tidal, and contemporary biofuels provide for more than a quarter—27.9 percent—of the world's electricity. In LMICs, the proportion of renewable electricity is less than one-fifth or 18.1 percent, whereas shares across countries vary from 1 to 100 percent. For example, Bangladesh's renewable energy share is 1.2 percent, Malaysia is 20 percent, Sri Lanka is 50 percent, the Democratic Republic of Congo is 99.7 percent, and Bhutan is 100 percent. A number of factors influence a country's share and potential of renewable electricity, such as geography, availability of resources, policy support, finance channels, and economic priorities. For various areas and countries, the installed capacity of power plants that generate electricity from renewable energy sources, measured in watts per capita, is displayed (figure 2.2). FIGURE 2.2. RENEWABLE ELECTRICITY-GENERATION CAPACITY (WATTS PER CAPITA) Source: Our World in Data based on International Renewable Energy Agency and United Nations World Population Prospects 16 Renewable electricity production per capita has doubled since 2010. Bhutan, which stands out with the highest electricity production per capita in the world at 3,026 watts, sells one hundred percent of their hydropower to neighbouring nations. China, the most populous country in the world, leads the renewable electricity sector. Its capacity per capita is 621 watts in renewable electricity, which is 2.5 times the global average. China has six times the renewable electricity capacity per capita as India. In Sub-Saharan Africa, renewable electricity capacity per capita is at 38 watts. As a result of ambitious renewable electricity legislation and falling solar and wind costs, adoption and share in renewable electricity have increased. In a decade, solar and wind electricity costs have decreased by 85 percent and 50 percent, respectively. Depending on the local context, renewable electricity has become the cheaper option in many places. The COVID-19 pandemic and the Russia–Ukraine war supply chain problems have reversed the cost decline. With an increase in commodity prices and transport and freight expenses, 25–30 percent more have been spent globally on utility–scale solar PV and onshore wind projects in 2021 than in 2019 (IEA 2021b). In India, for example, the impact of these increased costs has been exacerbated by: (i) a trade policy established to encourage domestic production of solar photovoltaic cells and modules; and (ii) an increase in the goods and services tax (GST) on renewable energy equipment and projects. These issues have jeopardized the sector's near-term prospects and necessitated more active government policy support to meet climate change objectives. SYNERGIES OF RENEWABLE ELECTRICITY AND ELECTROMOBILITY Increasing renewable electricity integration to the grid comes with challenges for the system's stability, such as boosting grid voltage—hampering performance of the connected power equipment like DTs by overloading— injecting harmonics, and so on. As a result of the intermittent nature of wind and solar generation, investments in equipment like inverters and storage are needed to reduce peak load, enhance power quality, and store excess power. Integrating storage with renewable electricity will increase implementation costs, which could impede the widespread deployment of renewable electricity in LMICs. Furthermore, using renewable electricity for EV charging requires additional grid infrastructure, leading to an increase in grid upgrade costs. Conversely, integrating a greater proportion of renewable energy sources into the grid for EV charging results in benefits like greater carbon dioxide emission reductions. As a productive use case for the electricity sector, e-mobility can be an anchor load—a new demand that provides the operators of renewable electricity generation and grid infrastructure with additional revenue and thus an incentive to invest (SuM4All 2021). Integrating the development of renewable electricity and e-mobility can also help minimize grid impact challenges: renewable electricity with a battery energy storage system (BESS) can act as ancillary support to the grid by storing energy during high renewable electricity output hours, supplying power during off-peak hours, and enhancing system reliability and resiliency. It also provides reactive power that helps with voltage control, thereby improving grid stability and minimizing technical and commercial losses. When using vehicle-to-everything (V2X)3 technology, batteries from EVs can act as BESS, supporting renewable electricity by storing power and reducing additional storage investments. The commercialization of renewable electricity has existed longer than that of EVs, with significantly greater investments and planning. Many LMICs have grid-scale renewable energy deployments and distributed renewable electricity projects with documented cost advantages over fossil generation. Integration and use of synergies of EVs and renewable electricity requires the development of an ecosystem capable of handling the increase of EVs and renewable electricity from the beginning of their transformation journeys. The technological maturity of renewable electricity, energy storage, smart grid, and electric vehicles can be a gateway for LMICs to increase the ELECTROMOBILITY AND RENEWABLE ELECTRICITY: DEVELOPING INFRASTRUCTURE FOR SYNERGIES • 17 efficiency of their transport and electrical supply chains through proper integration. The proper integration of renewable electricity with EVs and synergies with the grid on the technical, commercial, business model, and policy sides can be an opportunity for LMICs to optimize their grid modernization and charging infrastructure investments. With this, e-mobility can be a pathway to transition from a high carbon and unreliable electricity system to a low carbon, reliable system. This should enable countries to boost their ability to reduce GHG emissions, air pollution, and fossil fuel dependency, while simultaneously improving access to affordable and high quality power. OPPORTUNITIES FOR GROWING ELECTROMOBILITY AND RENEWABLE ELECTRICITY IN SYNERGY This section outlines opportunities at the intersection of both technologies and presents various EV charging business models integrating renewable electricity. While some of them are being prominently used, others are being piloted. The business models discussed examine different electricity generation models, different charging models, vehicle segments, and applications (figure 2.3). Five of the most relevant business models (figure 2.4) for LMICs are presented in more detail, and sequential to their potential priority. FIGURE 2.3. AREAS OF BUSINESS MODELS. Business Model Taxonomy Energy Model Grid Distributed Renewable Energy (DRE) Mini-grid Application Individual Intermediate Public Transport (IPT) Public Transport Freight Vehicle Segments 2W 3W 4W Buses Trucks (Light, Medium, Heavy) Charging Model Public Charging Station Home & Offices Battery Swapping Captive DRE Mini-grids 18 The business models are built on the experiences from LMICs moving forward with developing e-mobility. They comprise different parameters: (i) growth rationale that clarifies the need and competitive advantage of the business model; (ii) benefits of the model for the electricity and the transport sector; (iii) limitations of the model that could hinder its scalability; and (iv) suitability of the model to LMICs, determining its relevance and potential for deployment in an existing ecosystem. Eventually, for each business model, we recommend options that can support their development. The business models are ordered based on the affordability, scalability, and sustainability of developing specific e-mobility segments and associated charging infrastructure using renewable electricity. For instance, captive fleet4 charging with renewable electricity integration is a more affordable model compared to others like battery swapping, which involves high capital investment for battery swapping stations and renewable electricity integration. The order also considers gains in sustainability, for example, the electrification of public transport buses or commercial transport service vehicles have a higher potential for GHG mitigation—and thereby air pollution—than individual private vehicles. Commercial vehicles represent a relatively small share of approximately 20 percent of the global on-road fleet but contribute to a disproportionate share of on-road fuel consumption and emissions of nearly 70 percent. BUSINESS MODEL 2.1. CAPTIVE FLEET CHARGING WITH RE INTEGRATION FOR PUBLIC TRANSPORT E-BUSES, RIDE-HAILING TAXIS (2-, 3- AND 4-WHEELERS), AND FREIGHT VEHICLES FIGURE 2.4. FIVE DIFFERENT BUSINESS MODELS FOR E-MOBILITY AND RENEWABLE ELECTRICITY INTEGRATION. BM-1 Captive fleet charging with RE integration for PT e-Buses, ride-hailing taxis (2W, 3W & 4W) and freight BM-2 Green Public EV charging with RE and BESS (including kerb-side charging) BM-3 Utilities as integrated RE and EV charging-as-aService provider for homes and offices BM-4 Battery Swapping for light EVs Charging BM-5 DRE based Mini-/ Microgrid powering rural areas and EVs GROWTH RATIONALE • Globally, the shift to public transport (PT) bus systems is increasing with a drive for operational and financial efficiency and to increase fleet size with a transition to e-buses. Also, the ride-hailing freight services market is growing globally and making an economic case for their electrification. • Public transport e-buses, ride-hailing taxis, and freight vehicles having access to a dedicated depot in strategic city locations with ample spaces would provide an easy charging opportunity with renewable electricity integration. • As the push for freight electrification grows, warehouses make the most sense for charging points, considering the typical logistics profile of hub-and-spoke or point-topoint models, and vehicle layovers of ~5–6 hours during loading and unloading. ELECTROMOBILITY AND RENEWABLE ELECTRICITY: DEVELOPING INFRASTRUCTURE FOR SYNERGIES • 19 • Charging fleets with large battery capacities (for buses, trucks) demands more energy, which makes a case for integrating with renewable electricity to help reduce the load on the grid. • Big rooftops as well as ground space in depots and warehouses provide opportunities for renewable electricity integration for charging. However, given the size of fleets and the required high megawatt-level charging loads, especially in case of e-buses, captive renewable electricity—produced for an entity’s own consumption—alone may not be sufficient; additional supply from grid-connected installations, eventually off-site and with rights to use the grid for remote consumption may be needed and require open energy access BENEFITS • Support peak shaving (reducing load on the grid during peak hours) using renewable electricity with BESS for charging, thereby reducing the grid investment costs required to manage peak demand. • Reduce impact on the grid by reducing harmonics and voltage instability and thereby reduce losses in the system. • e-bus or e-truck batteries provide good stationary renewable energy power backup storage (as BESS) at lower costs given higher daily distance runs and battery utilization, thereby undergoing faster replacement. LIMITATIONS • High investment costs when using batteries to store renewable energy to charge a fleet overnight. • Scheduling a fleet to match charging pattern and renewable energy generation requires suitable locations to install renewable electricity on site or, alternatively, remote access to the national grid. SUITABILITY TO LMICS • e-buses provide higher GHG abatement per passenger-kilometer compared to other vehicle segments. Hence, they are often a high priority for LMICs to extend fiscal incentives and low cost financing from development finance institutions (DFIs) and climate funds. • Already a high share of private operators is managing intracity and intercity bus systems in LMIC cities, and the right initial support can bring investments in e-buses. • Apart from e-buses, ride-hailing taxis or fleets, and freight are also a prominent usecase for renewable electricity integration for captive fleet charging. RECOMMENDATIONS Government • Support deployment of fleet electrification through fiscal and non-fiscal incentives. • Provide additional incentives for charging captive fleets by renewable. • Support solar leasing (a business model where the client has the photovoltaic unit installed on site, yet the unit remains in the provider’s possession) to reduce capital expenditure for the client. • Allow renewable electricity units to transmit power through the grid to an off taker (open access). Fleet Operator • Open fleet charging location for private users (may be for some hours of the day) to add profit to the business. 20 BUSINESS MODEL 2.2. GREEN PUBLIC EV CHARGING INTEGRATION WITH RE AND BESS (INCLUDING KERBSIDE CHARGING) GROWTH RATIONALE • Public charging stations (PCSs) give visibility and confidence to EV users and help curb range anxiety. • Increasingly, more PCSs are getting integrated into existing matured commercial locations like fuel stations (intracity and highway), malls, restaurants, and parking. These locations typically have real estate for renewable electricity integration. • With many users resorting to planned home or office charging, rising PCS use cases, including kerbside charging, are moving toward quick opportunity or top-up charging. • Real estate space at PCSs and prevailing high electricity commercial tariffs makes a business case for renewable electricity integration. Depending on space availability at the site, 100 percent renewable electricity can be supported with a mix of onsite and remote generation with open access to the electricity grid or the right to trade in produced renewable electricity for deferred consumption. • Renewable electricity plus BESS combined with a grid makes PCSs greener. It also reduces both the electricity and demand charges. This, further integrated with smart chargers, will allow PCS operators to align pricing signals with utilities given ToU or ToD (time of use or time of day) tariffs and to drive EV charging users’ behavioral change. • BESS using repurposed batteries from EVs could further make its deployment with renewable electricity more economical. BENEFITS • Provides better grid stability and reliability by supporting peak load shaving (reducing load on the grid during peak hours), thereby reducing the required grid investments in equipment like inverters. • BESS at PCS will provide the necessary back-up power system at the time of grid failure/ outages and act as an ancillary support to the grid. LIMITATIONS • Requires considerable land or space for deployment, which is a constraint in urban areas. • Need for smart charging solutions to better manage multiple EV charging demands, which also calls for high investments. • Low utilization of PCSs affects the business economics. SUITABILITY TO LMICS • Most LMICs are in the early stage of EV adoption and have very low and scattered demand at their PCSs, posing a viability challenge for private investments without high capital subsidies. • Typically, the real estate cost in urban cities is high in LMICs, meaning that PCSs incline more toward fast charging than long-time parking and slower charging. • High voltage and high-power lines typically cost more in electricity infrastructure setup costs. As well, electricity commercial tariffs in LMICs are usually higher. The high sanctioned load for PCSs and lower utilization results in very high monthly demand charges. RECOMMENDATIONS Government • Provide capital subsidy to set-up public charging stations (PCSs) and kerb-side chargers. • Plan to make grids smart through deployment of smart meters and pre-paid meters to monitor and manage renewable electricity generation and EV charging. • Provide additional discounted tariffs to PCS deploying renewable electricity. Operators • Deploy smart solutions to manage uncontrolled EV charging at PCS. ELECTROMOBILITY AND RENEWABLE ELECTRICITY: DEVELOPING INFRASTRUCTURE FOR SYNERGIES • 21 BUSINESS MODEL 2.3. UTILITIES AS INTEGRATED RENEWABLE ELECTRICITY AND EV CHARGING AS-A-SERVICE PROVIDER FOR HOMES AND OFFICES GROWTH RATIONALE • The economics of renewable electricity and EV are becoming attractive for end users and their fast proliferation high grid impact. Progressive utilities have hence started facilitating their customers to improve energy efficiency, behavioral or automated demand response (i.e., devices which connect during low demand on the grid and disconnect during high demand) through use of ToU or ToD charging, and solar rooftop generation. • In many countries there is a growing trend of providing solar rooftop (SRT) as-a-service (long-term power purchase agreements or PPAs) to residential and commercial customers as a RESCO (renewable electricity service company) model. Private utilities are playing increasing roles in extending this service to their customers. • Many utilities in developed countries are extending the charging-as-a-service (CaaS) model to home and office customers; they are extending investment or rebates or offering both, and hence optimizing national cost on public charging infrastructure. • During high renewable electricity output hours, EV charging shifts—for relatively low power electric 2 wheelers and e-cars at home and offices—through appropriate ToU and smart charging can optimize further end user economics and also utility costs for grid expansion. • LMICs tend to have a high share of inverter and battery power backup systems at homes and offices. These storage assets can be leveraged to charge from captive solar rooftops and then support EV charging later in the day or night. Increasing new power backup systems would allow high loads (air conditioners, EVs) to be run through renewable electricity. • In LMIC rural areas, where rooftops are weak, the deployment of solar panels over utility poles and pooling the power can be used for charging EVs. BENEFITS • Managed and controlled charging can reduce impact of EV charging on the grid. LIMITATIONS • High investment required by utilities to deploy smart technological solutions to monitor real-time integration of renewable electricity and EVs. SUITABILITY TO LMICS • Many LMICs struggle with electricity availability challenges, and most public utilities are unable to bring enough investments in grid upgrades. The economics of solar rooftop systems that are connected to the grid are increasingly favorable to end users and there is rising adoption. The right fiscal incentives for solar rooftop investment and favorable net metering policy can bring mass distributed renewable electricity adoption and democratized investment in grid generation capacity. • Slow alternating current (AC) charging at homes and offices is the most-used type of EV (2 wheelers and cars) charging worldwide (more than public charging), offering additional load and revenue opportunity to utilities. • Utilities in LMICs can take on a higher renewable mix (via renewable purchase obligations or RPOs) and EV targets in their customer base. They can also leverage external investments in solar rooftops and EVs to their advantage through innovative tariff and settling mechanisms (like ToU/ToD/net metering) and driving appropriate demand responses, including EV charging, during renewable electricity rich time and avoiding bunching of grid peaks. 22 RECOMMENDATIONS Government • Mandate utilities by setting targets for renewable electricity deployments in the country • Incentivize users to use solar rooftops for charging EVs rather than exporting electricity to the grid. Utility/Operator • Provide solar rooftops to customers eligible for subsidies, which the government can support with a one-time subsidy; for example, in the agricultural sector, and provide further incentives for maintenance. • Reduce the power purchase rate of feed-in-tariffs for consumers to encourage the use of generated renewable electricity power in-house rather than exporting. BUSINESS MODEL 4.4. BATTERY SWAPPING FOR LIGHT EV CHARGING GROWTH RATIONALE • Battery swapping allows users to charge (swap the battery) quickly. • Battery swapping charging stations are solely allocated to batteries that will be used in swapping. This allows renewable electricity integration, including captive systems; fuel stations could be key locations to host battery swapping. BENEFITS • Is attractive for vehicle operators as swapping does not require the same amount of time as charging. • In commercial fleets, it can increase fleet utilization, improve logistics and delivery timelines, and save time. • Allows separation of batteries and EVs ownership, thereby reducing the upfront cost of EVs for the end user. • Provides an attractive business model for new energy operators through improved battery life, grid responsive charging, and renewable electricity integration. • Reduces investments in charging networks and centralizes electricity consumption. • Allows the use of batteries as storage (in a managed manner) and the ability to put power back into the grid. • Addresses space constraints in urban areas as multiple batteries can be stacked, using less space than parking for charging. LIMITATIONS • High investment cost, high degree of technical requirements, and high inventory of batteries. • Battery swapping stations demand high energy from the grid to keep the batteries charged round the clock. • Need for standardization of batteries (with different technologies) for interoperability without hampering technology upgradation. ELECTROMOBILITY AND RENEWABLE ELECTRICITY: DEVELOPING INFRASTRUCTURE FOR SYNERGIES • 23 SUITABILITY TO LMICS • Battery swapping fits best for commercial and fleet-operated electric 2-wheelers, 3-wheelers, light 4-wheelers (particularly commercial vehicles) which run a high daily distance and have certainty in the routes to allow optimizing the network of battery swapping stations. • Many LMICs have a dominant vehicle share of 2-wheelers, 3-wheelers, and light 4-wheeler commercial vehicles, with increasing fleet adoption in these segments. • The Asia-Pacific region dominates battery swapping applications in 2-wheelers, 3-wheelers, and light commercial vehicle segments, namely China, Japan, India, Indonesia, the Philippines, and others. RECOMMENDATIONS Government • Provision of capital subsidy and access to space at preferential rates to set up battery swapping stations and the extension of additional subsidies for integrating with renewable electricity. • Define interoperability standards to ensure safety and compatibility of battery packs. • Allow higher foreign direct investment in this segment to attract global investors when setting up battery swapping systems. • Provide a single point of contact for clearances and approvals for battery swapping projects. • Allow usage of waste lands, provide spaces or offer both to set up such battery swapping stations. Operators • Provide additional discounted rental/ leasing rates when using battery swapping with renewable electricity. • Use identical (singular type) of battery for swapping in multiples based on application. • Automate the process of battery swapping system for faster turnaround. GROWTH RATIONALE • Distributed renewable electricity-based mini or micro-grids are a solution for supplying electricity all day to many communities without adequate grid service. • The financial viability of most mini or micro-grids requires strategies to increase electricity sales. • Mini/micro-grids' need demand loads that can be time shifted to periods when renewable electricity is available, otherwise balancing the demand-supply will require substantial storage facilities. • EVs with large on-board energy storage can provide a base load to distributed renewable electricity mini or micro-grids and potentially help mini/micro-grid operators improve their business cases and expand energy services. • Distributed renewable electricity based mini/micro-grids can potentially be used as EV charging stations, like a battery swapping station that charges batteries during renewable electricity generation and then leases out these batteries to the EV drivers during operations. This would allow drivers to top up their EVs. • Distributed renewable electricity based mini/micro-grids can use sources like solar, biogas, or even hybrid sources of energy (like grid plus distributed renewable electricity). BUSINESS MODEL 2.5. DISTRIBUTED RENEWABLE ELECTRICITY-BASED MINI- OR MICRO-GRID POWERING RURAL AREAS AND EVS 24 BENEFITS • Provides access to affordable and reliable electricity and transport in underserved areas. • Reduces loss and wastage of farm produce because there is increased access to transport. • Provides a base load to minigrids improving the business cases for the operator and reducing costs for the end user. • Encourages rural entrepreneurship in providing renewable electricity to productive applications, like EVs for transport services, grain milling, rice hulling, cold storage, sewing machines, and food processing. • Ensures safety by providing power during night using battery storage. • Supports education by providing access to affordable transportation to schools or colleges. • Ensures seamless delivery of essential services such as healthcare, education (online learning), and internet connectivity, which have become vital owing to pandemic-like situations. LIMITATIONS • High investment costs for deploying minigrids and lack of financing support. • Requires regular local maintenance support (skilling) to keep minigrid workings for e-mobility applications. SUITABILITY TO LMICS • Access to both affordable and reliable electricity and transportation continues to be a challenge in LMICs. 238 million households will need to gain electricity access in SubSaharan Africa, Asia, and island nations by 2030 to achieve universal electricity access. • Diesel generator sets are used as an energy source, which is a highly polluting technology (including in island countries). • Distributed renewable electricity based minigrids offer an opportunity to power remote rural areas of LMICs. Minigrids can serve almost half of the total rural population—an estimated 111 million households. • Transport facilities are also limited in rural remote areas, and the modes of transport that exist are primarily 2-wheelers, 3-wheelers, and light 4-wheeler commercial vehicles. Hence this model will fit primarily for electric 2- and 3-wheelers but can be extended to light commercial vehicles depending on the size of the minigrid. RECOMMENDATIONS Government • Mandate that minigrids be considered part of formal energy planning processes and help support them with financial resources and incentives where required. • Support community-based minigrid projects as part of national rural electrification programs and poverty reduction efforts. • Provide financial and fiscal incentives to encourage minigrid development and keep electricity tariffs affordable. Fiscal incentives include customs waivers and tax breaks for investors. Financial incentives include grants, low-interest loans, and loan guarantees. • Provide incentives to independent power producers to sell surplus power from minigrids to the national grid. • Encourage small power producers with innovative business models to use minigrids to electrify rural communities and use them for charging EVs. Minigrid operator (private/ power utility • Encourage and incentivize charging of EVs during off-peak hours to help improve minigrid economics. • Support the deployment of EVs through rental models and create alternate livelihood opportunities for rural entrepreneurs. ELECTROMOBILITY AND RENEWABLE ELECTRICITY: DEVELOPING INFRASTRUCTURE FOR SYNERGIES • 25 ACTIONS FOR INTEGRATED DEVELOPMENT OF BOTH SECTORS The foundation to develop practical solutions for LMICs and address its barriers requires actions as enumerated in this section. These measures would address high investment costs, lack of economies of scale, silos of operation between stakeholders, and unreliable power supply. Governments: Drive renewable electricity and EVs demand and supply with a clear vision and a road map toward long-term commitments f Define e-mobility and renewable energy roadmaps with clear targets in adoption and production across different market segments. This will provide businesses with more certainty to plan. f Stimulate demand and develop the supply-side ecosystem through fiscal and non-fiscal incentives and the creation of funds for e-mobility market development. While most LMICs already have renewable energy roadmaps, higher renewable electricity targets in NDCs require defining for decarbonized e-mobility—extending green electricity across EV life cycle from mining, manufacturing, logistics, and charging to reuse or recycle. f Form clear institutional structures and allocate clear responsibilities between different ministries to collaboratively implement and invest in e-mobility and renewable electricity. f Develop standards and provide regulatory support for EV and renewable electricity integration. f Strengthen power utilities with a suitable structure to drive investments in efficiency, improving electricity access or off-grid development, and overall smart integration of renewable electricity and EVs. f Drive investments to strengthen domestic manufacturing units and efficient supply chains. Establish a circular economy to promote sustainable local supply chains. f Explore the potential of reducing import tariffs on key renewable electricity—photovoltaic modules, cells —and e-mobility battery components to accelerate adoption by lowering cost barriers. f Expedite transition by defining and enforcing end-of-life timeframes for old thermal power plants and vehicles. Align end user renewable electricity and EV incentives with scrappage of old technologies. To complement this, provide or extend facilities that would help operators to mitigate unintended environmental consequences. f Extend support, including guarantees, for early donor-based funding from DFIs and climate funds. f Establish new financial instruments and markets to reduce systemic risks associated with investments in renewable electricity and EVs, such as carbon markets. f Support commercialization of low cost climate financing for retail and business users to allow easy access to low cost finance. f Ensure that savings from renewable electricity and e-mobility—from high taxation, penalties or carbon tax on ICE vehicles, and reductions of subsidies on fossil fuels—are channeled to renewable electricity and e-mobility to support the growth of both sectors. f Incentivize the entire e-mobility value chain of local manufacturers, operators, and end users using renewable electricity to support sustainable deployment of e-mobility. f Provide additional incentives such as subsidies for charging stations be they public, private, or captive using renewable electricity and thus encourage service providers to adopt more and more renewable electricity. 26 Power Utilities: Start adding renewable electricity production capacities and support solar rooftops and smart charging solutions for customers, encouraging the use of renewable electricity for EV charging f Undertake strong network and system planning and define clear renewable electricity limits at distribution level, like peak shaving, reactive power (voltage) control, grid loss minimization, grid upgradation cost optimization by avoiding additional infrastructure costs —distribution transformers, low- and high-voltage lines. Such measures can be designed to help to leverage renewable electricity and EV benefits. Re-invest savings from aggregate technical and commercial loss reduction to reduce financial stress and to incentivize renewable electricity and e-mobility load management. f Support customer-friendly settlement incentives for solar rooftop systems that are connected to the grid. Introduce time of use (ToU) or time of day (ToD) tariffs to discourage EV charging during peak-hours and encourage charging during high renewable electricity output hours, maximizing the utilization of renewable electricity sources. Provide incentives for flexible charging and vehicle-to-everything from charge points or battery swap stations to incentivize shared use of resources. f Support the development of DRE based mini-grids, independently or with renewable electricity developers, to provide access and improve the reliability of electricity in remote areas. f Invest in making the grid smart through smart metering across elements and effective bidirectional communications and controls. This will need support from government and technology providers to drive improved energy efficiency, behavioural/ automated demand response, smart charging, and vehicle-to-everything services to customers and also to replace diesel generators as a back-up power supply. Use such technologies to enhance resilience and adaptive potential to respond to natural disasters brought about by extreme climate events. Manufacturers: Build affordable and locally suitable, high quality EVs (allowing renewable electricity integration) through efficient and clean supply chains f Build high quality EVs, following standards defined by regulators and accounting for user friendliness—in payments, communications— safety, durability, affordability, and the ability for end users to integrate renewable electricity to accelerate uptake of renewable electricity and EVs. f Provide high quality renewable electricity systems with the appropriate size or capacity and adherence to standards that support grid stability from harmonics to reactive power when integrating with EVs and operating as an ancillary support to the grid. f Develop efficient and clean supply chains by leveraging country resources, using renewable energy sources for electricity generation, and including re-used or recycled products to reduce the environmental impact from the production of EVs. Operators: Establish valued, scalable business models which can solve the affordability and productivity challenge f Install renewable electricity for different EV charging technologies—including battery swapping, portable charging, and fixed charging— with technical support from power utilities and financial support from government either state or federal. f Develop different charging-as-a-service scalable business models—subscription model, pay per use, charging per session—to provide EV charging at affordable rates by using renewable electricity. Financiers: Provide easy access to low-cost financing and scalable financing instruments f Include renewable electricity and EV related businesses in priority sector lending to enable access to financing for businesses and end users. f Dedicate green funds to grow the renewable electricity and EV market for innovative solutions. ELECTROMOBILITY AND RENEWABLE ELECTRICITY: DEVELOPING INFRASTRUCTURE FOR SYNERGIES • 27 f Provide finance for businesses to set up and scale EVs and renewable electricity production. f Provide consumer finance to purchase or lease EVs, batteries, and renewable electricity production units. f Work with insurance providers and businesses to mitigate technology risk. f Support the development of reuse or recycling to facilitate environmentally safe disposal of vehicles, batteries, and renewable electricity. RE Developers: Grow the renewable electricity mix with customers to achieve the best price for delivered electricity and high network efficiency with grid integration f Support the growth of renewable electricity deployments at affordable rates—with financial support from government or financing institutions—and provide high network efficiency with grid integration. f Develop distributed renewable electricity based mini-grids for off-grid customers and develop business models supporting the installation and maintenance of renewable electricity and e-mobility integration systems. Power Exchanges: Support infrastructure and price mechanisms that allow round-the-clock renewable electricity and EV integration f Develop appropriate grid-tied storage systems— pumped, battery, others—and appropriate mechanisms to build a market for round-the-clock renewable electricity supply. f Develop peer-to-peer (P2P) trading mechanisms to enable customers to unlock revenue by selling power from both renewable electricity and EVs. Notes 1. For more information on electricity access rates and other energy trends see: https://trackingsdg7.esmap.org/time. 2. Electric power transmission and distribution losses https://data.worldbank.org/indicator/EG.ELC.LOSS.ZS?locations=XO 3. Vehicle-to-everything is a technology that enables an electric vehicle to exchange energy and information with a connected entity of its environment; in this case, the entity can be an electricity grid, a local network (e.g., of a building), or a storage system. 4. Commercial vehicle fleets with regular and known duty cycles and a depot. References Bloomberg. 2021. E-Bikes Rule China’s Urban Streets: Hyperdrive Daily. https://www.bloomberg.com/news/ newsletters/2021-04-05/hyperdrive-daily-e-bikes-rule-china-s-urban-streets. BloombergNEF. 2022. Electric Vehicles Start Gaining Traction in Latin America. https://about.bnef.com/blog/ electric-vehicles-start-gaining-traction-in-latin-america/. Das, S. and Deb, S. 2020. Vehicle-Grid Integration: a new frontier for electric mobility in India. New Delhi: Alliance for an Energy Efficient Economy. https://aeee.in/wp-content/uploads/2020/10/Full-Report-Vehicle-GridIntegration-AEEE.pdf. Department: Statistics South Africa. 2021. National Household Travel Survey. http://www.statssa.gov.za/publications/P0320/P03202020.pdf. 28 Goldstein Market Intelligence. 2021. Asia Pacific (APAC) Two Wheelers Market Outlook 2024: Regional Opportunity and Demand Analysis, Market Forecast. https://www.goldsteinresearch.com/report/ asia-pacific-two-wheeler-market-report-analysis. IDCOL (Infrastructure Development Company Limited). 2019. Accelerating Green Buildings Transition in Bangladesh. https://idcol.org/bces-2019/assets/newsevents/knowledgepapers/Acceleratin%20Green%20 Buildings%20Transition%20in%20Bangladesh.pdf IEA (International Energy Agency). 2018. Electric power transmission and distribution losses (% of output) - Low & middle income. https://data.worldbank.org/indicator/EG.ELC.LOSS.ZS?locations=XO. IEA (International Energy Agency). 2021a. Global energy-related CO2 emissions by sector. https://www.iea.org/ data-and-statistics/charts/global-energy-related-co2-emissions-by-sector. IEA (International Energy Agency). 2021b. Renewables 2021: Executive Summary. IEA, Paris. https://www.iea.org/ reports/renewables-2021/executive-summary. IEA (International Energy Agency). 2022a. Global Energy Review: CO2 Emissions in 2021. IEA, Paris. https://www. iea.org/reports/global-energy-review-co2-emissions-in-2021-2. IEA (International Energy Agency). 2022b. Global EV Outlook 2022, IEA, Paris. https://www.iea.org/reports/ global-ev-outlook-2022. Our World in Data. 2021. Energy: Key Charts. https://ourworldindata.org/energy-key-charts. SuM4All (Sustainable Mobility for All). 2021. Electromobility in the Global South: An Equitable Transition Toward Road Passenger Transport Decarbonization. Washington, DC. https://www.sum4all.org/data/ files/05-electromobility_in_the_global_south_an_equitable_transition_toward_road_passenger_transport_ decarbonization_032322_v2_0.pdf. Sustainable Bus. 2022. Electric bus deployment speeding up in Latin America [TUMI E-Bus Mission #3]. https:// www.sustainable-bus.com/news/electric-bus-deployment-latin-america-tumi/ Toukam, N., Eva, S. and Mia, K.K. 2022, A Dozen Markets and Counting: The Opportunity for Two Wheel E-Mobility in Sub Saharan Africa. https://persistent.energy/wp-content/uploads/2022/02/Publication_The-opportunityfor-Two-Wheel-e-Mobility-in-Sub-Saharan-Africa.pdf. Sustainable Low Carbon Transport (SLOCAT). 2020. E-Mobility Trends and Markets https://slocat.net/wp-content/ uploads/2020/02/SLOCAT_2020_e-mobility-overview.pdf ELECTROMOBILITY AND RENEWABLE ELECTRICITY: DEVELOPING INFRASTRUCTURE FOR SYNERGIES • 29 KEY TAKEAWAYS f Railway transport must take a central part in the decarbonization of the transport sector, as it is more efficient in the use of resources than road-based modes. f Reaching the full potential of environmental benefits railways can bring also depends on the shift away from diesel toward low-carbon energy sources. f Railway electrification, the most traditional way to decarbonize operations, poses a significant economic challenge due to the considerable capital investment required. f Challenges related to railway electrification may be accentuated in countries of the Global South that often face financial constraints, and where the national power grids may need to be expanded considerably to cater for the additional demand from the operation of train. f The synergies between the energy and the railway sector may hold the key to bypass 'chicken-and-egg' paradigm and unlock the economic justification for such investments. f Railway electrification is only viable when sufficient electricity supply already exists or if electrification of railways and expansion of the grid can be combined so that these infrastructure systems promote wider transport and electricity access to the population and for economic growth. f Electrified railway services create a new and known demand for electricity and the Joint development of electrified rail and renewable electricity infrastructure can optimize investments in both sectors; this will require a holistic and integrated approach in planning, financing, and operations. EXPLORING THE POTENTIAL SYNERGIES BETWEEN RAILWAY ELECTRIFICATION AND GRID EXPANSION Marcelo Blumenfeld 30 3 RAILWAYS AS AN ENERGY-EFFICIENT AND HIGH-VOLUME MODE OF TRANSPORT As a vital part of the response to the climate emergency, railways offer great potential. This is because, despite being infrastructure heavy, railways are one of the safest and most environmentally sustainable modes of transport. Rail transport is highly efficient in use of space and energy, as it can carry 40 times more passengers per square meter, and it consumes only a third of fuel, relative to road transport, to carry a ton–kilometer. Rail freight produces between 75 and 85 percent less greenhouse gas emissions per transport unit when compared to articulated trucks. When we factor congestion, safety, and air quality, rail transport has the potential to reduce external costs of transport by at least 82 percent per passenger–kilometer, and 87 percent per ton–kilometer when compared to road modes (AfDB 2015). RAILWAY’S LOW CARBON ENERGY OPTIONS Powered with low carbon energy such as renewables, railway emissions are further reduced. Railway decarbonization can be achieved mainly in three ways: (i) electrification through catenary—overhead wires;(ii) a third rail through batteries; or (iii) with hydrogen fuel cell systems. Traditionally, electrification through catenary lines has been the primary choice for systems around the world. They provide, compared to other traction systems, substantial power at low noise levels and the ability to accelerate rapidly and operate at greater speeds. Yet, catenary electrification carries the highest costs associated with the construction of required infrastructure, where costs, depending on the local context, can range from US$250,000 in Egypt) to more than US$2 million in the UK) per track–kilometer (Blumenfeld et al. 2020). In Europe, the European Commission (EC) found a range between €100,000 and €1.5 million (EC 2018). Where full electrification of the line is unfeasible, alternative traction solutions can offer ways to decarbonize railway operations without the need for trackside infrastructure. One of these options is the use of battery-powered trains. Ever lower charging times are making battery systems more applicable to the needs of railway services. Yet, these systems offer a limited range of approximately 100 kilometers (Railway Technology 2020) and may be better suited for urban or suburban passenger lines. As with the use of electric batteries for the decarbonization of road transport, standards and regulations for recycling, reuse, and disposal of batteries are needed to mitigate environmental risk and ensure efficient use of resources. Finally, hydrogen as a fuel source for hybrid systems has gained prominence as an eventually clean and low carbon solution. These systems can become a competitive alternative, because they have a significantly higher range than battery electric trains due to the higher energy density of hydrogen. Other than batteryelectric systems, hydrogen fuel cells do not carry social and environmental concerns regarding resources and end-of-life management. However, hydrogen fuel cell trains are under development and are challenged by the requirements of heavier freight traffic. They also require an entire supply chain in place with local production capability as well as the logistics and infrastructure to supply the fuel to filling locations along the railway network. While all three technologies—full electrification, battery power, and hydrogen power—come with zero carbon emissions at their point of use, they will, eventually, need to be coupled with electricity production from renewable sources to support the transport and energy sectors’ decarbonization further. CHALLENGES AND SYNERGIES OF DIRECT RAIL ELECTRIFICATION Even though electric batteries and hydrogen fuel cells may become solutions in specific cases, direct electrification of rail is likely to remain a central approach for its decarbonization. Electrified railways can generate greater traffic densities, which in turn improve the financial viability of those investments. Yet, funding of investments in electrified rail seems to be at the core of a chicken and egg situation. On the one hand, railway electrification is more financially justifiable where grid and production capacities already exist. On the other hand, a certain level of electricity demand is needed to make investments in grid expansion and ELECTROMOBILITY AND RENEWABLE ELECTRICITY: DEVELOPING INFRASTRUCTURE FOR SYNERGIES • 31 capacity financially viable. This points to the potential synergies between the investments required for energy and railway infrastructure. Combining both protagonists under a holistic view may open doors to develop production capacity and unlock grid expansion and the attendant overall social and economic benefits. In many countries, both sectors, energy, and railways, are vertically integrated under public ownership. Within each sector, separate entities overlook planning, construction, and operation of the systems. However, energy and transport should be planned together as they are practically interdependent. This requires, in the first place, close coordination between the public entities in charge of electricity and the railways and enabling private operators to invest and participate in the development. In the energy sector, a known and stable demand in electricity for rail could attract private investments from independent power producers (IPPs) to produce and sell in the relevant ranges of capacity and voltage. Adequate legal frameworks are essential prerequisites to unlock maximum potential of synergies that enable public and private stakeholders to collaborate effectively, to build on existing and potential demand, and to support national plans for expansion of both networks, rail and electricity. When railways and energy are understood and planned jointly, the large investments required can be more easily justified and made attractive to public and private initiatives. Therefore, a cohesive and synergistic approach across sectors is a need rather than a choice. EXPERIENCES IN THE GLOBAL SOUTH Countries of the Global South are showing a renewed interest in building modern standard gauge railway (SGR) networks under the African Union’s flagship Agenda 2063 to increase connectivity in the continent. These plans would also contribute to the decarbonization of transport by their lower emissions per transport unit, However, to further reduce their emissions, low carbon energy must increasingly replace the use of diesel and of fossil fuel generated electricity. In Africa, for example, most railways run on diesel. The continent is far from its future vision for 2063 of a continental network of electrified railway corridors. Less than 15 percent of the almost 80,000 kilometers of railway lines in Africa are electrified, spread across only eight countries (UIC 2021). If South Africa is not counted, then only six percent of the railways are electrified. The electricity sector is facing its own challenges. According to data from the World Bank, less than half of the population in Sub-Saharan Africa has access to electricity (World Bank 2022a). As a result, should some low-income countries with high railway traffic densities—ton–kilometer per route–kilometer—such as Guinea and Liberia want to electrify their railways, it could require up to almost 80 percent of their existing electricity generation capacity (BP 2020; UIC 2019). These cases of gaps in energy and railway infrastructure highlight the potential to address the development needs of entities together. A good example of how the synergies between sectors can be explored is illustrated by the construction of the Ethio-Djibouti Railway (EDR), which opened in 2018. The planning of the fully electrified 760-kilometer line connecting the two countries was conducted in parallel with works on new hydroelectric plants to increase the national power generation infrastructure. The new electrified railway required additional generation capacity, and the government saw the potential to expand the national electricity production capacity and grid to cater to both the country’s development needs and the new rail connection. This guaranteed that the national grid would be able to supply enough power over the entire length of the line, comprising 19 stations in Ethiopia and three in Djibouti. In return, the power plant gained a new consumer, with a constant and sizable electricity demand. In total, the investment of almost US$4 billion was considerable for the Ethiopian economy and was not free of contention. However, the large capital costs should not be seen as a barrier when considering the vast benefits that electrification brings, as Tebebu Terefe, Chief Safety Officer at Ethio-Djibouti Railway stated in an interview to inform this article. First, the increased demand in gigawatt-hour reduced overheads 32 and the unit cost of electricity, making the operation of railways, and other uses of electricity, more cost efficient. EDR now pays as little as Ethiopian 0.79 birr (US$0.015) per kilowatt-hour, which makes operations largely competitive against diesel powered lorries. Second, the savings in fuel costs and the reduced fuel dependency are considerable and were the principal line of reasoning for EDR’s railway electrification program. The line now carries more than two million tons of freight per year, including the important economic export of coffee. Each of the 1,900 freight trains operated this year has replaced 53 lorries, which equates to savings of more than 114,000 tons of carbon dioxide emissions in the country and reduces the other important externalities associated with road transport. Finally, the physical displacement of railway assets can help bring the grid to rural areas where access to electricity is needed and where the low demand in electricity from rural communities alone would not justify investments in grid expansion. Ethiopia is not alone in the list of countries prioritizing investment in appropriate infrastructure for the long-term benefits that it provides. In Africa, Tanzania is rapidly advancing its plans for a standard gauge railway network of more than 2,700 kilometers electrified lines at a total cost of US$6 billion. These run alongside the country’s Tanzania Rural Electrification Expansion Program (TREEP), a US$200 million initiative with support from the World Bank, which is dramatically widening access to electricity (World Bank 2022b). Both countries, Tanzania and Ethiopia, have placed their projects under a holistic national plan that allowed them to secure investments that build on the synergies between both sectors. With the new railway lines, not only will carbon emissions be cut, but freight costs are expected to be reduced by 40 percent (IRJ 2021). In Asia, India has more than doubled the number of electrified route–kilometers, and it is on track to have its network—one of the largest in the world, —fully electrified by 2024 (CORE 2022). Coupled with the electrification program, Indian Railways are partnering with solar plant operators to construct additional renewable capacity in and along railway spaces and feed the energy directly into the traction power. With 51,000 hectares of vacant land, Indian Railway has potential for an estimated 20 gigawatt of solar electricity, and the will to make use of it. ELECTROMOBILITY AND RENEWABLE ELECTRICITY: DEVELOPING INFRASTRUCTURE FOR SYNERGIES • 33 References AfDB (African Development Bank). 2015. Rail Infrastructure in Africa: Financing Policy Options. African Development Bank, Abidjan. https://www.afdb.org/fileadmin/uploads/afdb/Documents/Events/ATFforum/ Rail_Infrastructure_in_Africa_-_Financing_Policy_Options_-_AfDB.pdf. Blumenfeld, M., Hillmansen, S., and Burrows A. 2020. Enhancing shift towards Sustainable Freight Transport in Asia and the Pacific. UN. ESCAP, Bangkok. https://www.unescap.org/sites/default/d8files/knowledge-products/21_July_2021_RailDecarbonisationReport_Final.pdf. BP (British Petroleum). 2020. Statistical Review of World Energy 2020. British Petroleum, London, 2020. https:// www.bp.com/content/dam/bp/business-sites/en/global/corporate/pdfs/energy-economics/statistical-review/ bp-stats-review-2020-full-report.pdf. CORE (Central Organisation for Railway Electrification). 2022. https://core.indianrailways.gov.in/. European Commission. 2018. Assessment of unit costs (standard prices) of rail projects (CAPital EXpenditure), Final Report. https://ec.europa.eu/regional_policy/en/information/publications/reports/2018/ assessment-of-unit-costs-standard-prices-of-rail-projects-capital-expenditure. IRJ (International Railway Journal). 2021. Electrical testing begins on Tanzania’s new standard gauge railway. https:// www.railjournal.com/infrastructure/electrical-testing-begins-on-tanzanias-new-standard-gauge-railway/. Railway Technology. Bombardier Talent 3 Battery Train. https://www.railway-technology.com/projects/ bombardier-talent-3-battery-train/. UIC (International Union of Railways). 2019. Adoption of new sustainable mobility commitments. UIC, Paris. UIC (International Union of Railways). 2021. RAIL ISA Statistics. https://uic-stats.uic.org. World Bank. 2022a. Access to electricity (% of population). https://data.worldbank.org/indicator/EG.ELC.ACCS.ZS. World Bank. 2022b, Changing Lives and Livelihoods in Tanzania, One Electricity Connection at a Time. https:// www.worldbank.org/en/news/feature/2022/06/28/changing-lives-and-livelihoods-in-tanzania-one-electricityconnection-at-a-time. 34